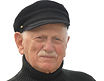

Fishing about and about fishing
MARINE FARMING
“As a primary goal, aquaculture development should conserve genetic diversity and minimize negative effects of farmed fish on wild fish populations, while increasing supplies of fish for human consumption”.
(U.N. Code of Conduct for Responsible Fisheries, 1995).
.
Fish farming or aquaculture was defined by the Food and Agriculture Organization of the U.N. (FAO) as “the farming of aquatic organisms, including fish, crustaceans, mollusks, and aquatic plants”. Marine farming, or marine aquaculture, or mariculture can be defined as “farming of marine organisms”. It also implies some sort of ownership of the cultivated stock (Bailey et al, 1996), although not necessarily of the area over which the marine farm is anchored or staked. Sea or ocean ranching, therefore, doesn’t fall under this definition, since the “seed” fish grown in hatcheries once released are not owned, until captured. Aquaculture, including marine farming, has become a mass provider of animal protein, employment option for poor farmers and displaced capture fishermen, solution for marginal lands and water resources, and as exporter – a foreign currency earner. With marine and inland capture fisheries unable to satisfy a growing demand for some popular fish species, such as for example, salmon, cod and shrimp, as well as trout, tilapia and carps, respectively, it has also become their complementary source.
Aquatic foods have high nutritional quality, contributing on the average 20 percent or more of per capita animal protein intake for almost 3 billion people, mostly in developing countries. Fish is also the world’s most widely traded foodstuff and a key source of export earnings for many countries with particular significance for small island states and, as far as fish farming goes, for countries in the South and Southeast Asia.
History. Aquaculture has been practiced by mankind since times immemorial. Five thousand years ago, Chinese villagers trapped carp in artificial lakes that formed when flooded rivers receded. Ancient practice of carp culture in China was described in the 5th century BC, while the Egyptians might’ve been cultivating fish a thousand years earlier. Also the ancient Romans grew oysters and, most probably, fish, already 2,000 years ago. Around 600 A.D., the Menehune of Hawaii built a huge fish pond on Kauai, as the legend goes, by erecting overnight a 900-foot-long wall out of lava rock, (Cufone, 2008).
Most of the ancient fish farming was based on capture of young stages and transferring them to ponds and sea enclosures to grow. This could be done, for example, by fencing off tidal areas toward ebb tide, which arrested fish movement seaward and facilitated their collection during the outgoing flow. Another method, practiced till the present in some places, is letting fish enter open enclosures during seasonal floods to feed and grow there, and fencing them off before the water recedes. Fish are kept in those enclosures to grow, or collected and moved elsewhere. Such types of culture can be seen even today, for example, in Italy’s “vallicultura” and in SE Asia.
All these and other early variants of fish farming have been of the extensive type. Extensive fish farming implies that fish are not crowded much beyond their population density in nature and are not or almost not artificially fed. Thus, extensive fish farming hardly affects natural habitats. Environmental problems appeared only with intensification of the production.
While the ancient fish farming was based on eggs/larvae/juveniles collected from nature, in a true full circle aquaculture they should be produced on the farming premises. First to close the growth circle was a German farmer in the 18th century who fertilized trout eggs with their sperm and hatched them in tanks/ponds.
During most of the 20th century, marine fisheries and fish farming were developing in parallel as separate industries with little market interaction. By and large, each produced different species and had respective traditional consumers. But during recent decades, things have been changing on the fish market. Now many consumers buy fresh or smoked salmon, sea-bream, oysters, or frozen shrimp, many without knowing, and some without caring, whether they were caught in open sea by fishermen, or grown in ponds, mollusks farms, or floating cages. Presently, farming of some 15 species of marine finfish is in its starting phase or expanding, each according to the progress made by researchers and fish farmers.
Notwithstanding, capture fisheries and fish farming are inter-related and to a great extent overlapping in their ecology, economics and social impacts. Both interact in several manners and often co-exist in common ecosystems. This essay approaches marine farming development, recognizing the fact that our whole civilization has been based on man-modified ecosystems both on land and in water, and that further modifications are required in view of the badly mishandled needs of the expanding human population and the sustained destitution of many.
Statistics. In 2007, the total world fisheries yield was over 140 million mt, of which some 90 million was produced by capture fisheries and over 50 million by aquaculture, most of it in the developing countries, with China leading with some 31 million mt (FAO, 2010). In 2006, the world consumed 110.4 million mt of fish, 51.7 million mt originating from aquaculture. The earth’s population is forecast to reach 8.32 billion in 2030. If capture fisheries production (92 million mt in 2006) and the non-food uses of fish (33.3 million mt in 2006) remain constant, aquaculture needs to produce 80.5 million mt by 2030 in order to maintain the current per capita consumption of 16.7 kg. That is, 25 years from now, aquaculture will need to produce 28.8 million mt more per year than its current annual production (FAO, 2008).
The recent development of aquaculture. During the last 4 decades, fish farming has expanded to meet the soaring global demand for seafood. Both on land and in the sea it has undergone tremendous quantitative development, and soon will be overtaking capture fishery in the global supply of food fish (Tacon and Halwart, 2007).
Consumption of fish, both wild and farm raised, has doubled since 1973, with most of this increase in developing countries. But as the demand for fish rises, wide populations of both marine and freshwater species are unable to produce significantly increased yields, because of stagnant or declining catches. The total marine fish yield has reached 90 million mt a year, a yield that after a period of rapid growth and despite increasing demand has remained mostly level for the last decade.
Since the later years of the 20th century, salmon has become the main finfish farmed in cold waters, milkfish, shrimp, and yellowtail - in South Asia’s and the Far East’s ponds, cages and lagoons, and seabream and seabass - in cage farms all over the Mediterranean. Atlantic salmon, a fish exotic in Chile and on the west coast of North America has been farmed there since the mid 1990s. A relatively late comer to the salmon industry already a decade ago Chile produced more than 100,000 mt/year, and earned in 2006 over US$2.2 billion. This followed a 15-year development during which production expanded by an incredible 2,200 percent. Between 2003 and 2006 Chile’s export earnings grew by an average 22 percent per year, challenging Norway as the world’s top salmon producer.
Unfortunately, it has been suffering during the last couple of years from massive outbreaks of salmon disease that caused considerable decline in production and earnings. Cod farming, already beyond the R&D stage, has become a reality mainly in Norway, the UK and Denmark. Also farming of halibut and turbot is becoming commercial.
The tuna grow-out industry, pioneered in Australia, towards the end of the 20th century has expanded also in the Mediterranean and the Central Atlantic Ocean’s coastal waters. The practice of tuna on-grow (fattening) in cages, however, cannot be considered a real farming. In fact, it consists of creaming off of young fish from the stock years before they had any chance of spawning, and fattening them for half-an-year, or so, before harvesting. This procedure must negatively affect wild stocks of the most sought after tuna species. In Japan, in a procedure that shouldn’t affect wild tuna stocks, it is the fry that is caught to be fattened at a fish farm.
Recently, however, successes were reported of closing the whole life cycle of tunas, i.e., holding breeding stock, making them spawn and fertilize the eggs, on-growing larvae into fingerlings, etc. (Anonymous, 2008; Fiske, 2008). This, if fully implemented could increase very much the production and relieve the pressure on the wild populations. The snag is that tuna are grown on other, forage fishes, and if pellets are used, these would contain high share of fishmeal produced of "industrial", as a rule, small pelagic fishes, of which several kilogrammes are needed to produce one kg of tuna.
The dilemma of growing demand and sustainability. Worldwide, both governments and international and regional organizations define their marine policies as aimed at achieving sustainability in aquatic ecosystems and, particularly with respect to aquatic life and fisheries resources. At the same time, it is widely recognized that the present production of fishery products from the wild and from the existing aquaculture would not be able to match the increasing food needs of the expanding human population.
The U.N. defines…”sustainable development” as development “that meets the needs of the present without compromising the needs of future generations... to be achieved through …balance between environmental integrity, social development and economic development…”. According to FAO, sustainability entails the notion of progressive development, which has no negative effect on the environment and on the future of the resource concerned (Caddy and Griffiths. 1995).
But, “sustainable development” suffers from different, often incompatible interpretations by economists, sociologists, environmentalists, and various stakeholders (Ben-Yami, 2006; Sharp and Hall, 2004). This argument ranges from the extreme “nature-first” conservation approach to a “development and business first” approach. The former is about maintaining of or returning to marine ecosystems as close as possible to their “virgin” or pre-industrial state (Ben-Yami, 1996). The latter is about extraction of fishery resource in the most profitable way, now, (Goodland and Daly, 1996).
Constraints. It is important to bear in mind the various constraints that may impede further development of marine farming.
Although growing sea fish in floating cages may be, under some conditions, less costly than on land, the marine fish farming industry has been focusing on raising species, which when supplied from capture fisheries, fetch relatively high prices. While it indeed may serve as a mass protein provider, its product is intended only for those who can afford the price. Hence, repeating cases of salmon market gluts. They are good and popular fish, but many potential consumers can only afford to pay for them prices that the salmon producing-processing-marketing system cannot live with (Ben-Yami, 1999).
According to FAO, aquaculture would keep growing so that the 2010 world’s total fish production may reach some 140 million mt. While the supplement must come from farming, some 30 million mt of marine fish landings would go for fishmeal needed to feed, apart from poultry and cattle husbandry, and inland aquaculture, all the predatory fishes that are grown in marine cages. This is a nagging problem, all the more that the feed alone may account for some 60% of total production costs (Forster, 2008). Processing and marketing technologies are advancing, and no doubt, some of the today’s “industrial” fish will be made fit for human consumption and fetch prices that would make their reduction to fishmeal uneconomic. Availability of fishmeal, therefore, may become a constraint; hence, quite appropriately, vegetable surrogate feeds are investigated and here and there already applied.
Species that feed at lower levels of the food pyramid, like, carp, milkfish, tilapia (Gur, 1997 and private communication) and gray mullets and, according to information from Israel, also gilthead seabream, can be grown on reduced fishmeal or vegetable diets (Kissil and Lupatsch, 2004). Nonetheless, fish farmers still prefer to use feeds that incorporate some fishmeal, lack of financial motivation being one reason, inertia – another (Ben-Yami, 2004-b). Tilapias are basically freshwater vegetarian fishes, widely cultured throughout the world, with some species showing euryhaline behaviour. Recently Malta University reported on trials aimed at growing tilapia both in sea cages and in tanks with sea water.
Other problems confronting the marine farming industry are environmental concerns, public resistance to genetic engineering, bioaccumulation of various chemical substances by fish grown in polluted waters, frequent outbreaks of stock-devastating diseases, and proliferation of parasites.
Outlook. While markets and consumers get the fish they need, fishing industry strategists and also many fishermen look with wary eye on the ongoing expansion of marine fish farming industry. Although farmed sea fish cannot be sold cheaply, their regular supply prevents wild-fish prices from going up, even off-season to compensate a fishery for poor landings. Together with reduced quotas and subsidies, and several impoverished stocks, this may lead to significantly unfavorable consequences, and even shrinking of the affected capture fisheries. On the other hand, an increase in landings of wild fish of farmed species or market-wise equivalent may affect prices of the farmed fish with negative economic consequences for the farmers.
With this bad news come also a bit better ones. Firstly, where this happens, marine aquaculture may represent a way out for investment capital off the capture industry, and a second line of defense to displaced fishermen by providing employment. Secondly, fish farmed in the sea might resist unfavourable environmental changes in water better than wild fish. This, because if the organisms that form the natural food base of the fish in the wild are seriously affected by such changes, the food base of the wild fish stock would be reduced. Their displacement or disappearance may cause wild population's migration or starvation or both. Farmed fish are supplied with their food whatever is the temperature and salinity of the surrounding water and would suffer only at the extremes of their physiological survival range. All this may make redundant fishing capital and low-income fishing people consider, respectively, investment and employment in the marine farming industry, and in particular – in offshore cage farms, where seamanship, sea legs, boat-operation, and net-making and net-mending skills represent important advantages.
According to FAO, the maximum wild-capture fisheries potential from the world’s oceans must’ve been reached, thus almost any further growth in the world’s fish supply will come from fish farming (FAO, 2006). The rising demand and technological developments that have been fueling the explosive growth of fish farming, have stimulated what is considered by many to be the most hopeful trend in the world's increasingly troubled food system (Hailweil, 2008; Halwart et al. 2007; Ryther and Bardoch, 1968), although targeting at the more affluent consumer (Ben-Yami, 1986). In any case, the mankind is consuming more and more seafood, not so much owing to increase of consumption per capita, but rather due to the continuing growth of the world’s population. In this context both, in terms of demand and of supply, the share of Asian aquaculture is overwhelming.
The commonly proposed solution for the problem of satisfying the demand for fishery products is both to improve the management of exploited fish stocks, and to further the development of commercial fish farming – a process, which is already going on at accelerating pace. There is a continuum between open-access fishing and intensive aquaculture, along which fishing rights and property rights develop from vague to almost absolute (Anderson, 2002), often within shared habitats. However, such developments represent a focus of an earnest dispute on a global scale. This, because they affect inter-related processes within the domain of marine ecology, of economics and of social systems of coastal communities and other people involved in fishing, fish farming and fish processing and marketing.
The spiraling rate of fish farming development, however, has produced also undesirable "side effects” that could have been mostly avoided, would the necessary attention be paid timely and adequate money and efforts duly invested. Notwithstanding, as long as it can produce fish at prices people are ready to pay (Asgard et al, 1999), aquaculture will keep expanding, especially, if wild marine and freshwater yields won't grow, while demand increases.
Feed and energy. Presently, small, forage fish account for some 37% of the world’s sea fish yield, and 25-30 million mt of them are reduced into fishmeal and oil, of which close to half is used for poultry and cattle fodder. In 2006, aquaculture consumed approximately 3.06 million mt or 56 percent of world fishmeal production and 0.78 million mt or 87 percent of total fish oil production with over 50 percent of fish oil going to salmon farming. Between 1992 and 2006 the content of fishmeal and fish oil used in fish feeds has tripled on the expense of the poultry sector, which gradually reduced its reliance on fishmeal. Besides fishmeal or fishmeal-based diets, an approximate 5 to 6 million mt of low-value/trash fish are used as direct feed in aquaculture, and notably in tuna cage culture.
FAO (2008) doesn’t expect any significant increase of fishmeal production, all the more that the fishmeal usage in animal production sector, particularly poultry, will continue to decrease in the coming years. Furthermore, it is expected that a proportion of fishmeal and fish oil used in fish feed will be replaced by vegetable-based protein and oil, and that feed management efficiency is going to be improved. Additionally, price level is bound to affect aquaculture production, especially, when the demand for aquaculture products is outstripping the supply, and fish prices soar so that even inefficient farms make money (ibid; Jackson, 2009).
The expansion of salmon culture in three continents – Europe, North and South America - has resulted in increased demands for feed fishes. Recently, farming of cod – another piscivore – has become the focus for massive development efforts. The magnitude of the farming of piscivorous species competes with the previously predominant culture of tilapia, carp and catfish that utilizes mainly vegetable or algae-based feeds (FAO, 2008).
Apart from the tuna that’s mainly fattened on small pelagics, predatory fish grown in ponds and cages are usually fed with mixed feeds with varying share of animal protein. Fishmeal is very concentrated (some 60% of protein) and normally about 4.5 kg of raw fish are needed to produce 1 kg of fishmeal (Jackson, 2009).
Piscivorous fishes such as salmon and trout must be fed with animal protein; hence fish farming industry has become a major consumer of fishmeal. However, the world resources of fish that can be economically utilized for reduction are finite and as mentioned above, with the right market conditions and appropriate technology, some of them could be used for human consumption and might increasingly leak away to canning, sushi-type and fresh-fish markets. Thus, the presently spiraling growth of aquaculture rather sooner than later must face fishmeal supply constraint.
Altogether, the use of forage fish for fish feed has become a worrying situation. The existing management efforts focus on groundfish and large pelagics, rather than on forage fish, and little is known about their role in the marine ecosystem both as grazers and as food for seabirds and marine mammals, and what the true relation between their natural and fishing mortality is. If and when the demand for forage fish by human food market increases, some of the fishmeal industry and, consequently, also the fish farming industry, may find themselves facing feed prices that may preclude feasible operation. Also, according to Halweil (2008), although raising seafood like oysters, clams, catfish, and tilapia is many times more efficient than cattle breeding, a growing scarcity of fish feed may jeopardize future expansion of farming piscivore fish, like salmon and cod.
If such shift occurs, one would expect increasing reduction of the fishmeal contents in the various feeds and pellets, improving feed-production and feeding-in-cage technology. Today, at a rather conservative feeding coefficient of, say, 4:1, with feed containing 25% of fishmeal, 5 kg of raw fish is needed to produce 1 kg of farmed fish weight. (Jackson, 2009). Only with much higher and hardly practiced fishmeal content of 50%, 10 kg of raw fish for 1 kg of farmed fish weight would be needed.
Reduction of fishmeal content in the feed of some other fishes grown in cages that are feeding in wild on invertebrate fauna, like seabream, is also indicated, in view of satisfactory performance of vegetable proteins (Kissil et al, 2004). Although replacement of fishmeal by vegetable (soy, etc.) meals depends on their respective prices, a steadily increasing amount of the food supply for farmed fish could be coming from soybeans and other vegetable products.
Additional options could be to amplify the preparation of feeds from non-food by-products, such as fishing by-catch and discards, fish-processing offal and fishmeal made of industrial non-food fishes (Alverson et al. 1994), as already up to one-third of fishmeal is made from various fishery wastes (Forster, 2008-b). Another way is using a mixture of poultry by-products and vegetable oils, such as worked out by a Malaysian team headed by Rossita Shapawi (Shapawi et al., 2007).
Claims that those are the fish-farming feed requirements that detract forage fishes from becoming food fish are misplaced, for comparable amounts of fishmeal and oil are going into margarine, cattle feed and chicken feed. Fish farms compete for access to that supply with other, non-fisheries industries, a competition that influences prices for both the fishermen, who catch the small forage fish and for the fishmeal-and-oil industry.
The very processing and transportation of the fishmeal and the forage fish, often overseas, require energy, which reduces the economic efficiency of the whole process. Thus, when the energy costs and other costs of contemporary fish farming in marine floating cages are examined, it turns out that e.g. salmon aquaculture may be even less efficient than extremely inefficient high seas salmon trolling (Folke et al., 1994). On the other hand, the farmed fish consume far less wild fish protein per unit of weight growth than are consumed by wild fish, which must spend lots of energy to chase down their prey. According to Asgard et al. (1999), for example, 10 kg of capelin produces some 4.6 kg of farmed salmon, while the same weight of capelin would only produce 2.0 kg of wild salmon.
Better utilization of both the fish farming area and the energy in the feed would make the industry more environment friendly. But, to introduce and invest in innovative industry practices that may help to create a shift toward progressive fish farming, there’d also be a need for a fundamental change in public attitudes. This includes a willingness to prioritize fish that are lower on the food chain and sensitivity to environment friendly practices. But can consumers today be mobilized to modify the aquaculture industry as they pressured tuna fleets to adopt more dolphin-friendly practices in the 1980s?
Problems. In recent decades, marine farming of seaweeds, invertebrates and finfish has become a main player in national waters of many countries. Therefore, in coastal waters, whether those facing open sea or those in enclosed and semi-enclosed habitats, any fisheries management must cover both capture fisheries and marine fish farming, as it must take into account also all other major stakeholders and participants in activities that affect environment in the managed area.
The "integrated coastal zone management" (ICZM) is supposed to take care of such problematique. Born as a tool to prepare nations for a possible rise in the level of the sea, it is now being applied by governments to harmonise, with their own policies, the often-conflicting interests of the various stakeholders. By definition ICZM has to get involved, at least partly, in fisheries management. The level of its involvement depends on both, the local fisheries, socio-economic and physical conditions, and the respective government policy. (Ben-Yami, 1998; 2005).
Coastal mariculture and in particular fish culture in floating cages, much because of its impact on coastal ecosystems and capture fisheries and on the socio-cultural fabric of fishing communities, have been under substantial pressure of criticism on the part of environmentalist organizations and activists, of commercial and recreational fishermen and other concerned bodies and individuals, and of the ensuing restrictions and regulation. Some, even, like Volpe (2004) insist that the salmon industry is clearly a net-loss proposition, whether viewed from the ecological, social or economic perspective. This criticism cannot be waived aside as that of some of the extreme critics of capture fisheries. It must be seriously examined and considered with the view of finding solutions to the negative effects of the mariculture.
Parasites and diseases spreading in the densely stocked fish cages and coastal marine ponds often cause mass mortalities and great financial losses to the fish farming industry, while escapees from cages may carry them to the vulnerable wild populations. While sea cages protect farmed fish from predators, pathogens can move or be carried both ways. A sea cage thus becomes an “unintended pathogen factory” (Frazer, 2008) or receptacle. According to some reports, each year around two million farmed fish escape in the Atlantic. Of fish farmed, unlike the quantitatively less important wild seabass and seabream, cod and to some degree salmon create large wild stocks of major commercial importance. Hence, the capture fishery is looking with a wary eye on the impact of infected runaway fish on their wild brethren, and on the possibility of interbreeding of farmed varieties with wild stocks. Also, sea-lice spreading from cages to wild fish may increase their lice burdens and cause wild fish to decline (ibid).
While fish infected in the cages have better chance to survive, owing to veterinarian care and various treatments, wild populations are more vulnerable. Massive infections of wild fish can be avoided only by ensuring that they do not share water with farmed fish, either by locating sea cages very far from wild fish migrational paths and feeding/spawning grounds, or through the use of closed-containment systems (ibid).
Fish farms with tons of feed being released into the cages create algae-promoting conditions similar to pollution from cities and terrestrial farms. The situation is reversed with oyster farms, because oysters are filtering animals. These farms provide water-cleaning benefits and support coastal economies. They also make better oysters: a farmed oyster is plumper, sweeter and prettier than its wild cousin. Hence, farming planktivorous, herbivorous and omnivorous freshwater fish like grey mullet, tilapia, carp and catfish, and mollusks like oysters and mussels is environmentally sounder. But the money is in the piscivores, like tuna, salmon and cod. The need to feed them with small whole fish or fishmeal is putting pressure on populations of wild forage fishes, which makes the fish-farming industry competing with wild carnivores over their limited resources.
With time, more problems materialized that, while not markedly slowing down the development, produced negative effects. Such has been, among others, the denuding of mangrove areas by the expanding shrimp farming in Latin America and in the south and southwest Asia, which has allegedly affected the catches in the adjacent artisanal fisheries and their communities, and more recently, was blamed for at least part of the damage caused all over the Indian Ocean by the 2004 tsunami.
Poorly run land-based fish farms, floating fish cages, and feeding pens release into the environment unutilized protein, nutrients and fecal matter creating wasteful and often noxious pollution. According to some estimates, a fish farm with 200,000 salmon releases nutrients and fecal matter roughly equivalent to the raw sewage generated by 20,000 to 60,000 people and Scotland's salmon aquaculture industry produces the same amount of nitrogen waste as the untreated sewage of 3.2 million people - just over half the country's population (Halweil, 2008). Residual feed and bio-products pollute protected waters causing increased turbidity, and over shallow bottom - desertification. Complaints on the part of bathers and the tourist industry have forced some cage farms in the Mediterranean and the Red Sea to transfer their operations ashore (Ben-Yami, 2002).
Effect of fish diseases and other environmental problems on cage farming. Concentration of fish farms both, land-based and marine, along coastline, especially in or near enclosed or semi enclosed marine areas as for example, Norwegian fiords, Scottish lochs, Southeast Asia, China and Japan coastlines, and North American estuaries, is allegedly harming the coastal marine habitats and spreading diseases and parasites to wild fish populations, of which salmon seems to be particularly hit.
Parasites are a serious problem associated with densely populated fish cages. Caligid crustaceans, such as sea lices Lepeophtheirus salmonis and Caligus spp, are parasitic copepods that attach themselves to fish, marking their hosts with ugly lesions that lessen their market value, stunt their growth and weaken their resistance to diseases. Found on wild fishes, they are now common among Atlantic salmon grown in cages in Norway, Scotland, Chile, and North America. But now there’re serious concerns that cage farms of salmon set on the migrational routes of wild salmon increase the risk of infection of the latter by sea lice originating from the fish cages.
The most harmful disease, spreading over cage farms affected by sea lice, is the infectious salmon anemia, or ISA. A highly contagious virus, ISA can be lethal to fish although it does not affect humans. The ISA virus is fast to spread over whole regions causing heavy financial losses and reduction of salmon production. One consequence is operators having to shut farms and processing plants and axing jobs. Also, diseases may leak from marine cages and pens and spread to wild populations – (Diamant et al., 2007). ISA was first discovered in Norway in 1984. In mid-2007, it turned up in Chilean waters, and within 2 years devastated the country's farmed salmon industry, which was forced to curb production by half and shed up to 4,000 jobs.
Another concern is the residual materials from the drugs used by fish farmers to fight parasites and diseases and the pesticides used in agriculture. Their amounts may be substantial in view that, apart from the residual veterinary products in the agricultural effluents flowing into the seas, the fish farming industry alone spends on drugs an estimated $1 billion a year. All those, including toxic substances affect inshore and coastal fish, including the farmed ones, in various ways, sometimes causing various distortions and growth arrest in juveniles growing in polluted waters (Saiki et al, 1992; Palawski et al, 1985).
Anthropogenic pollution and effluents affect both wild and cultured species inshore and in coastal waters. Serratia marcescens, bacteria commonly found in the guts of people and animals is killing off corals and can also kill fish (Patterson and Potter, 2003).
Unpredictable and uncharacterized impact of climatic, weather, and tectonic events presents unquantifiable threats. The first decade of this century has seen unprecedented impacts on aquaculture in Aceh (Indonesia), Bangladesh, China and Myanmar, which have all suffered from severe natural disasters (FAO, 2008).
On the other hand, fish farmed in the sea might resist environmental changes in water, including the effects of climate change, such as temperature variations, better than wild fish, which depend upon their natural forage organisms. Forage species, however, may be more sensitive to such changes than the fish themselves. Their scarcity or disappearance may cause their predators’ migration, starvation or both. Farmed fish are supplied with their food whatever is the temperature and salinity of the surrounding water and would suffer only at the extremes of their physiological survival range.
Aquaculture and the capture fisheries. Allocation of marine areas for fish and shellfish farming had become a problem when farms were established within traditional fishing grounds. While fish cages shouldn’t be and need not be placed over prime or traditional fishing grounds, not everywhere regulations prevent ill-conceived offshore aquaculture projects and provide for their removal. The accelerated development of aquaculture is calling for specific regulation and for allocation of marine areas where fish farms can be established, which willy-nilly must affect some traditional and other fisheries, through redistribution of access to fishing grounds essential for setting-up of the fish-farms. Notwithstanding, some of the claims regarding displacement of fishing grounds by aquaculture seem exaggerated, at least when it comes to open sea. The area taken by fish farms over exploitable fishing grounds is significant fishing-wise in a relatively few cases.
Apart from complaining that marine fish farms are expanding over fishing grounds, many commercial fishermen, oppose fish farming in floating cages, because of the associated influx of nutrients into the environment and of biological effects of densely populated fish farms on the habitat and wild stocks through broadcasting of parasites and viral diseases over wild fish populations and possible genetic effects due to massive escapes of farmed fish into open sea. The possible effects of fish escapes from cages in areas where massive marine farming is practiced, as in the N. Atlantic, at both sides of the Pacific, in Chile’s waters and in the Mediterranean, have been widely studied and discussed (Ben-Yami, 1999; Dempster et al., 2007; Halweil, 2008; Standal and Bouwer Utne, 2007).
All this taken into account, growth of supply of fish from marine and on-land farming is a must, as long as mankind keeps its excessive proliferation and the demand is growing, and properly managed fish farming can not only help feeding an expanding global population, but also relieve the pressure of fishing on wild fish populations (Halweil, 2008).
PROSPECTIVE DEVELOPMENTS
The question is not how to prevent aquaculture from harming the marine environment, but how to develop aquaculture that’s environment friendly.
It is beyond question that, in spite of its shortcomings and contention with other stakeholders, marine fish farming is here to stay, develop and expand. Restraining its growth is not in the best interest of the protein-hungry mankind, and any such attempts, including those flying the banner of sustainability, will eventually prove futile. The world is in need of a progressive development approach (Issar, 2008 & 2009) that would focus on how marine fish farming could expand while mending its ways, so that its present and future structure and development would cause minimum environmental and social damage and maximum social and economic benefit.
Regulation. For this purpose, it must be recognized that regulation of marine farming both, by national authorities in coastal waters, and by international agencies in the open ocean is crucial. The choice of technology and culture methodologies cannot be left to private enterprise alone, for it would obviously be looking for the most profitable, rather than for the most environmentally and economically feasible techniques. The same goes with respect to the contaminants content in the farmed fish flesh. This points to a greater role for aquaculture certification and standards and to labelling, such as exist, for example, in organic and local foods sector (Halweil, 2008). Progressive regulation should force the marine farmers to design and apply ways and means that while maintaining economical feasibility would be environment friendly.
Unfortunately, legal mechanisms available to legalize and regulate ocean fish farms in national and international waters are few and far between. One problem is that different national agencies have jurisdiction over different aspects of activities in seas and oceans, such as commercial shipping lines, fisheries, naval restrictions, coast guard and safety issues, pollution control, sand end minerals dredging, oil and gas explorations, offshore wind-energy farms, and extraction facilities like rigs and pipelines, etc. Many countries have not yet decided on specific standards for establishment and managing offshore fish farms, and/or on which agency or agencies should govern such fish farms, and how.
All this can make regulation, including site allocation and licensing, as well as enforcement, a highly controversial political issue (Bailey et al, 1996) with conflicting stakeholders’ interests, especially with regards to small private and community-operated enterprises versus corporate interests, and fish farming versus (commercial and recreational) fishing interests. On the top of it are powerful environmental lobbies that, rightly or wrongly, partly or totally, resist mariculture. Therefore, the very existence and character of state policies or their absence, as well as the level at which they’re implemented are critical to the development of marine farming, (Phyne, 1996).
Much of the conflicts could be avoided, if mariculture developers would follow such procedures as those appearing in the 303-pp ‘Guide for the Sustainable Development of Mediterranean Aquaculture 2 (Ben-Yami, 2010, IUCN, 2007).
Notwithstanding, marine aquaculture will keep developing, within the existing legal frame, or if non-existent, unrealistic, or ineffective – without. The extent and character of this development would be in accordance with the extent and character of market demand, while adjusting to its changing preferences, such as growing demand for organic products, attention to environment-friendly production procedures, etc.
TECHNOLOGY
Storm-proof cages. Submergence represents the most logical solution to assuring survival of fish cages and tanks in stormy weather, and is a must in case of net cages that are not fitted in stiff framing. The main principle of the submersible cages technology is that upon the approach of stormy weather, the cage is submerged, desirably by remote control, to a safe depth at which it is not affected by sea waves when the sea is rough. Several variants of submersible cages have been designed and some are in use, their shortcoming is that to be effective, they are not built as large as some of the surface-floating ones.
Some fish species suffer from various forms of decompression sickness, if they’re forced to ascend too fast from a deepwater submergence, cod, for example, may burst its air bladder. Therefore, the ascent of submergible cages containing such fish up to the surface should be quite gradual. This technology would keep improving and developing towards automatic submerging and surfacing of the cages by means of weather sensors and remote control. Another solution is to keep the cages permanently submerged and serviced by automatic feeding systems, and making them surface only for harvesting (Forster, 2008).
The special needs of servicing, mechanization and automation in marine fish farms would bring about design, vessel conversions, and construction of specialized sea-going work-boats (ibid), with size, form and functions fitting the design, location and character of the individual fish farms and their cages.
Containment. Closed-containment technology can prevent escaping into the ocean of both, fish that may affect wild stocks, and water that would spread parasites and thus also diseases. Closed containment farming requires floating tanks that must combine ship-type strength and seaworthiness with maximum mechanization and automation of servicing by remote control. They may be anchored or secured to piles, or even free floating in oceanic currents (see “Ocean drifters”, below). Fish in containment tanks won’t be able to escape and would grow without risk of attacks by marine mammals, which is common in regular net cages, while filtrating or other pollution preventing system would minimize environmental effects.
Abandoned oil rigs, artificial reefs and islands. One idea is to develop finfish aquaculture industry using open net pens or submersible cages in association with derelict oil rigs. Around such rigs, as with any offshore natural (reef, sea-mount) or man-made structure, would develop a multi-species biotope, including micro-fauna and other planktonic organisms that might serve as extra food for the fish in cages. Artificial islands and their immediate surroundings (Ben-Yami & Nir, 1990) could serve as bases and sites for marine fish farms. Seeding bio-filtering organisms to create their colonies/reefs in the vicinity of cage farms can reduce the deterioration of water quality due to waste and residual food from the cages (Ben-Yami, 2004).
Expansion of aquaculture over additional species. Dried sea cucumber fetches extremely high prices on the growing Chinese affluent markets as a delicacy and an aphrodisiac. So far, all the supply of sea cucumbers has come from collecting from the wild inshore and in shallow coastal areas, which brought about substantial impoverishing of the resource. Efforts towards stocking and even farming sea cucumbers have been going for years (Ito and Kitamura, 1998; ICLARM, 2002). Recently, in the Philippines sea cucumber was cultured for the first time in fishponds in polyculture with shrimp, following a successful experimental breeding that produced juveniles for stocking both, in fish farms and in the wild (Rosario, 2008). In the future, more and more commercially valuable finfish and invertebrates will be cultured, following the ongoing research and experimentation.
Polyculture. Fish in cages utilize the energy of only part of their feed. The remainder and the waste are flushed out of the cages. Hence, the idea to cultivate species that live at different stages of the food chain on the same or neighbouring site. For example, keeping salmon, mussels and kelp in the same system may enable the feed to be fully utilized, because the mussels and kelp can absorb it in various ways and grow on the feed not consumed by the fish and their waste (J.N.Galloway – Internet). Another solution could be keeping non-fed omnivorous fish in the lower level of “double-bottom” cages, where they can feed on what’s sinking through the “floor” of the upper level where carnivorous fish are intensively fed (Porter et al, 1996).
Genetic engineering. Genetically modified organisms (GMOs) represent a controversial issue in agriculture. Significant research has gone into producing GMO fast-growing fish and transgenic aquatic organisms, and some are ready to be placed on the market and under the U.S. Food and Drugs Administration’s (FDA) scrutiny (NY Times, June 25, 2010). However, the global debate on their possible risks to human and environmental health and in part inspired by moral values, sometime rather sanctimoniously, is continuing at such a scale that, according to FAO (2008), it is unlikely that in the short term GMOs will make a significant contribution to meeting the future demand for fish (Purdue News, 2010; The Center for Food Safety, 2009).
Farming for bio-fuel production. One way in which marine farming can do good service to the global environment is to grow marine plants, including algae, for bio-fuel production (Forster, 2008-b). Since this could be done on the basis of waste, effluents, and other coastal pollution, on which the plants would grow, the total environmental benefit should be considerable.
Aquaculture off-shore. There are many reasons why the marine farming sector is aiming for the open sea, in spite of some claims that open net cages flush pollution, disease and parasites into the ocean, adversely impacting wild fish supply, and the health and sustainability of the oceanic habitat. However, the water quality here is better and the flow rate is higher than within narrow or partly enclosed inshore areas, and the temperature is more stable – factors that improve the growth conditions and the quality of the fish flesh. Open water systems farther offshore should be less of a concern, because the waste dilution process is more efficient, so that cages sited over deep ocean water, especially where oceanic currents prevail, may have little impact on the surrounding water quality or the ecosystem, in general. Also the available evidence suggests that in open ocean aquaculture there is no measurable nutrient loading in the effluent. However, offshore aquaculture creates also its specific set of complications, such as problems with rough weather conditions, allocation and enforcing farm siting rights, and servicing the cages far offshore.
The pro-and-contra dispute is ranting, and in view of the evident necessity for further expansion of marine farming, people are expecting national and international regulation, and are looking at technology. Unsurprisingly, some rather futuristic ideas have popped up.
Open-ocean mussel farming
A new pilot-project run by the Marine Biological Laboratory (MBL) in Woods Hole, Mass., and coordinated by Scott Lindell, launched four experimental mussel farms to test blue mussel farming offshore Massachusetts and Rhode Island. It uses technology developed at the University of New Hampshire, consisting of anchored longlines suspended 10 m below the ocean surface, holding biodegradable “socks” filled with mussel seed, and relies upon the oceanic currents for the supply of food to the growing mussels. This development may lead to the creation of a multi-million dollar sustainable industry involving local fishermen, existing shore-side infrastructure and an underutilized natural resource.
Ocean drifters. A team at the Massachusetts Institute of Technology reported designing a giant remotely operated motorized cage, which is supposed to be carried by ocean currents, in a controllable manner. The idea is to keep cages drifting within a limited area or along a desired path, so that they can be filled with fingerlings, for example in Florida, and drift in the Gulf Stream for nine months to reach Europe with a market-sized crop. The MIT’s drifter-cages would be fitted with low-power hydraulics or electric self propulsion to allow them to remain within the current and stay away from bad weather. Not being anchored over a fixed spot, there’ll be little benthic impacts. MIT has just completed in Puerto Rico the first round of experiments with the Self Propelling Fish Farm Prototype. One of the research scientists’ visions is an advanced fish farm that can “think for itself”. Such fish farms will be able to float to more optimal locations when required and submerge in the sea when exposed to rough weather. Even though this vision is unlikely to be a reality for at least 10 or 15 years, most of the technology for fish farms drifting in the open sea is already available (Robson, 2008).
Sea ranching. The term sea or ocean ranching is used here to describe the attempts at increasing the output of natural and fishery ecosystems through the stocking of juveniles into vast open areas (Isaksson, 1994), but mostly in inshore and coastal waters. Such stocking with fingerlings or juveniles of edible invertebrates and finfish is limited to species that are either strictly local or seasonally returning to the locality, and depends on aquaculture technology that controls spawning and/or egg and larval development, until the animal grows to a stockable size.
Culture and stocking of juvenile lobsters and sea cucumbers in the coastal wate+++rs of Galapagos was proposed as a way to conserve the islands’ ecosystem, in view of heavy extraction of these species by local fishermen (Ben-Yami, 2001).
A group of Israeli fish farmers and scientists put forth an idea of fish ranching, which they call “virtual cage technology for farming of fish”. They propose to train juvenile fish in hatcheries to associate acoustic and visual signals with food. Trained fish are released to the sea to grow in nature; however, to perpetuate their response to the signals they’re fed periodically from floating platforms. At harvest time the acquired response would attract the fish to collection sites to be trapped and harvested selectively using a computerised vision system. This technology offers an alternative to cage farms and is almost neutral environmentally. It should be beneficial to fishermen and consumers, and may complement coastal cage culture and in some places replace it. It would save investment in expensive offshore cage farms and in floating growth-and-service installations and feeding technology, and save on running expenses for servicing labour (Zion et al, 2007). This, rather futuristic development requires working only with fishes that tend to stay in one locality, grow fast enough in nature, and have a good value on the market.
MARINE FISH FARMING ON LAND
Carrying sea fish farming ashore opens several interesting options. One is to regard the fish to be only one component of an integrated system in which also other organisms are grown. Such aquaculture could be based on algae or other plants, and designed to develop in a way, which could exert less pressure on the environment (Forster, 2008-b) and even enhance it. In addition, the option to grow sea fishes in land-based installations or in ponds represent a solution for situations where sea-borne farming in cages is excluded for environmental reasons or due to successful pressure of other stakeholders (Ben-Yami. 1999; 2002).
Closed, integrated systems. A promising development is integrated
aquaculture, for example, growing seaweed, salmon and mussels in a single installation. The seaweed and mussels live and grow on the waste from the fish. This principle may be further integrated in a closed system where the fish are fed and produce nutrient-rich waste. Solid fish waste may be removed to feed, for example, worms bred for bait. Water with dissolved nutrient waste may then be piped or otherwise released to feed water-filtering shellfish and plants. Such system can be fully closed as far as fish are concerned with very little additional water needed and no waste released (Moss, 2009).
Dr. Yonathan Zohar developed at the University of Maryland fish farming technology for the urban environment. His special self-contained and densely populated fish tanks are filled with recirculating water, kept free of waste by bacteria cultured on plastic plugs. Fish swim in tanks in water free of nutrient waste and are not exposed to toxic contaminants and poor water conditions. They can grow faster — eight to nine months compared with 14 to 16 months — and at much higher densities. The toxin-free fish can be marketed as clean, and conditions can be tailored to fit the fish. Farming sea fish in such land-based closed systems may also prove economically more feasible than in floating cages in open sea (Zohar, 2009; Zohar et al, 2008). On the other hand, however, important elements of this technology may well find applications also in marine fish farms where closed containment fish tanks are employed at sea. Most recently, such self-contained super-intensive shrimp culture system was developed at Texas A&M by Dr. Tzachi Samocha, (2009).
Fish farming in arid areas. In some arid and semi-arid areas, as for example in Southern Asia (Petr, 2003), the Middle East (FIS - Newsletter <newsletter@fis.com> Oct 04 2007) or in the Israeli Negev, saline, often warm ground water is available. While such water may be too salty to support agriculture, it may be suitable for aquaculture, starting with algae of species valuable for diverse end uses, including medicinal ones, and ending with marine and euryhaline invertebrates and finfish (Kraft, 2007). Development of such aquaculture may become an effective alternative livelihood for people living in arid areas. Undoubtedly, any such development would require substantial investments in research and infrastructure, choice of or devising methodology and, because of the need for strong technical support - an area-wide extension service. Studies and experimental culture projects have yielded a range of marine and freshwater finfish species that can be successfully grown in the desert, including tilapia, barramundi, sea bass, striped bass and red-drum.
In recent years this option has been attracting attention of international organizations, and quite rightly so, in view of the fact that semi-arid and arid areas constitute more than 40 percent of the global land area. Such areas are home to nearly one third of the global population, the bulk of who live in developing countries and represent some of the poorest people in the world. ‘Arid aquaculture’ in dry lands would present a supplement or a substitute to traditional crop farming and livestock rearing, might mitigate the consequences of desertification and improve nutrition through diet diversification.
CONCLUSIONS
General
Both, in spite and because of the fact that capture fisheries and marine farming in some areas are sailing on collision course and in others in a shaky and often volatile co-existence, the future development and management of both industries should be comprehensively designed, planned, and executed within the context of ecosystem management. Both industries are essential and even crucial for supplying the humanity with protein, in view of the pressures of the ever increasing demand. Constraining mariculture development is a non-starter, for it must lead to obstructing growth of fish supply and even to reducing fish production. Therefore, the easy way out of trying indiscriminately to curb their future development and even to cut down both, under the banners of “sustainability” and "precautionary principle" is unsustainable. Nonetheless, such course of action is assisted by alarmist and fallacious "scientific" articles published only due to inadequate peer-review process (Ben-Yami, 2003; Longhurst, 2007), and hence propagated by the general media always happy to quote sensational "science”.
A progressive approach (Issar,op.cit.), therefore, must be adopted and implemented for the development of marine aquaculture so that it is environment-friendly and socially acceptable, and that it can live in sustainable coexistence with marine capture fisheries within their common ecosystem, benefit fishing people and other fishworkers, and not less important, the consumers. The following conclusions can be suggested:
(1) It is imperative that, for the sake of sensible, rational and efficient management, both industries, marine aquaculture and capture fishery, fall in their shared countries under the same legal and enforcement authorities. This will enable rational allocation of farming sites and fishing grounds, abatement of conflicts, and maintaining an equitable modus vivendi.
(2) It is important to recognize that there’re no “one-size-fits-all” solutions, and there’s no panacea for every problem encountered by fish farmers, fishermen, and authorities in charge of fisheries (capture and aquaculture) management throughout the world. Every environment, every fishery, every fish species, and every site impress their specific conditions and requirements.
(3) Notwithstanding, a general approach in the spirit of progressive development should be common to all marine fish farms and, for that matter, the whole mariculture industry. While such approach takes for granted the need for and inevitability of further expansion of marine fish farming, its future management and development should be based also on the following principles:
(3.1) farm sites should be selected and allocated so that they do not take up traditional fishing grounds, especially those of small-scale and subsistence fishermen;
(3.2) farm sites should be selected and allocated so that they are not on the way of massive, seasonal or annual, migrations of wild fish populations;
(3.3) marine fish farms should be operated according to clear and strictly enforced rules aimed at minimizing their effect on the neighbouring habitat;
(3.4) marine fish cages should be designed to prevent escapement of fish and, especially in shallow waters, to contain or re-cycle residual feed;
(3.5) application of drugs and chemical substances, such as pesticides, hormones and antibiotics, should be regulated, monitored and the ensuing rules strictly enforced;
(3.6) coastal aquaculture farms should not be established upon environmentally sensitive, in particular mangrove-covered coastal areas;
(3.7) marine farms should not import and breed exotic species, except if established by specific research that their eventual escape into the wild would not be detrimental to the local biota and ecosystem;
(3.8) fish farmers should, as far as feasible, minimize the use of small forage fishes and fishmeal made of them.
(3.9) in fishery management, the "precautionary principle" should not be blindly applied with "fish first", whenever the managers find themselves in doubt as to what to do. It should be borne in mind that the resource is managed for the sake of people, and that the "doubt" in the expression "when in doubt" may still carry a tendency to lean either in favour of the resource or of the humans, and that the " taking no risk whatsoever" strategy may be detrimental to human societies;
(4.5) ecosystem management must pay the due and appropriate attention to reduction and elimination of all sorts of pollution and its sources, to prevention of inshore habitat destruction and to recovery of essential habitats for fish reproduction and nurseries, such as mangrove forests, wetlands, reefs, etc. On the other hand, it must take into account changes and fluctuations in marine climate, its physical and chemical phenomena, and the various biological factors that affect recruitments and natural mortality in wild fish, and to a lesser extent in the farmed ones..
***************
The needs of the world population, the constraints of the wild fishery resources, and the new problems arising in the wake of the development of marine farming, require a major shift in the prevailing interpretation of the term sustainability and, hence, in the approach to the management and development of all sectors of marine fisheries. The gist of the new and progressive approach is to fulfill two main objectives: 1 – increasing the supply of marine products to the people; 2 – sustain the fishery ecosystem on all its components: the producers and their communities, the fish and their habitat, and clean water in the sea.
**********************************************
REFERENCES
Alverson, D.L., M.H. Freeborg, S.A. Murawski and J.A. Pope. 1994. A global assessment of fisheries bycatch and discards. FAO Fish.Techn.Pap.(339). (FAO, Rome). 233 pp.
Anonymous. 2008. Grow your own bluefin. Fish.News Internatl. 47(12):16-17.
Anderson, J.L. 2002. Aquaculture and the Future: Why Fisheries Economists Should Care. Mar. Resour. Econ. 17:133–151.
Asgard, T., E. Austreng, I. Holmefjord, and M. Hillestad. 1999. Resource efficiency in the production of various species. In: Svennevig, N, H. Reinertsen, and M. New (Eds.). Sustainable Aquaculture: Food for the future? Pp. 171-183. (A.A. Balkema, Rotterdam, Netherlands).
Bailey, C., S. Jentoft and P. Sinclair (Eds.). 1996. Aquacultural Development: Social dimensions of an emerging industry. (Westview Press, Boulder, CO, USA). 281 pp.
Ben-Yami, M. 1986. Aquaculture: The importance of knowing its limitations. Ceres, 19 (4):15-19. FAO, 1986.
Ben-Yami, M. and Y.Nir. 1990. Ecological aspects of artificial islands – the Israeli context). In: Gallil, B. & Y.Mart (eds.). Studies of Mediterranean coastal margins of Israel. Collection of Lecture Abstracts. (Inst.Nat.Conserv., Fac. of Life Sci., Tel Aviv Univ.). 3rd Session, 1 p.
Ben-Yami, M. 1996. Ecological and socioeconomic aspects of the expansion of Nile Perch in Lake Victoria. Pp. 95-110 in: Meyer, R.M. et al (Eds). Fisheries Resource Utilization and Policy. Proc. World Fisheries Congress. Theme 2. Oxford & IBH Publ.Co., New Delhi.
Ben-Yami, M. 1998. ICZM and the role of scientists. In: Gallil, B. & Y.Mart (eds.). Mediterranean coastal margins of Israel. Collection of Lecture Abstracts. (I.O.L.R. – Natl. Inst. Oceanogr.). P.33-36.
Ben-Yami, M. 1999. A collision course with aquaculture. World Fish. 1999 (1):6.
Ben-Yami, M. 2001. Managing artisanal fisheries of Galapagos. A Consultancy Report – 07-01-2001 – 03-02-2001. (Charles Darwin Research Station; Ecuador Fisheries Department).
Ben-Yami, M. 2002. Sea cage fish farming decline. World Fish. 2002 (10):10.
Ben-Yami, M. 2003. "Nature" stumbles. World Fish. 2003 (8):8
Ben-Yami, M. 2004. Will science help cage farmers clean up their act? World Fish. 2004 (1):18.
Ben-Yami, M. 2004-b. Fishmeal. Posting on FISHFOLK (Fishfolk@mit.edu).
Ben-Yami, M. 2005. Managing the conflict alone. World Fish. 2005(1):6.
Ben-Yami, M. 2006. The meaning of sustainability. Part 1, World Fish. 2006 (5):6; Part 2, World Fish. 2006 (6):6.
Ben-Yami, M. 2007. Marine fish farming: Problems and remedies. World Fish. 2007 (10):6.
Ben-Yami, M. 2010. Marine farming – solution or delusion? World Fish. 2010 (3):6.
Caddy, J.F. and R.C. Griffiths. 1995. Living marine resources and their sustainable development – Some environmental and institutional perspectives. FAO Fish.Techn. Pap. (T353). (FAO, Rome). 167 pp.
The Center for Food Safety, 2009. Genetically Engineered Fish. Internet information. office@centerforfoodsafety.org
Cufone,M. 2008. Ocean Fish Farms and Public-Resource Privatization. The Amer.Prospect, November 24, 2008. (Internet).
Dempster, T., Moe, H., Fredheim, A. and P. Sanchez-Jerez, 2007. Escapes of marine fish from sea-cage aquaculture in the Mediterranean Sea: status and prevention”. CIESM, 2007. Impact of mariculture on coastal ecosystems. CIESM Workshop.
Diamant, A., A. Colorni and M. Ucko. 2007. - Parasite and disease transfer between cultured and wild coastal marine fish. CIESM, 2007. Impact of mariculture on coastal ecosystems. CIESM Workshop.
FAO, 2006. The state of world fisheries and aquaculture (FAO, Rome).
FAO. 2008. Opportunities for addressing the challenges in meeting the rising global demand for food fish from aquaculture. Committee on Fisheries, Sub-committee on Aquaculture. 4th Session. Puerto Varas, Chile, 6 - 10 October 2008
FAO. 2010. FAO fisheries global statistics: capture and aquaculture. http://www.fao.org/fishery/statistics/en.
Fiske, E. 2008. ALLOTUNA duplicates bluefin breeding success. editorial@fis.com. www.fis.com
Folke, C., N. Kautsky and M. Troell. 1994. The Costs of Eutrophication from Salmon Farming: Implications for Policy. J. Env. Manag. 40(73):182.
Forster, J. 2008. Emerging technologies in marine aquaculture. In: Rubino, M. (Ed.) 2008. Offshore Aquaculture in the United States: Economic considerations, implications & opportunities; pp. 51-72. Pre-publication copy. (U.S.Dept. of Commerce, NOAA, Silver Spring, MD).
Forster, J. 2008-b. Broader issues in the fish farming debate; pp. 245-259. In: Rubino, M. (Ed.) 2008. Offshore A|quaculture in the United States: Economic considerations, implications & opportunities; pp. 51-72. Pre-publication copy. (U.S.Dept. of Commerce, NOAA, Silver Spring, MD).
Frazer, L.N. 2008. Sea-Cage Aquaculture, Sea Lice, and Declines of Wild Fish. Conservation Biology, (Published Online: Dec. 10, 2008); http://www3.interscience.wiley.com/journal/120122721/issue
Goodland, R., and H.Daly. 1996. Environmental Sustainability: Universal and Non-Negotiable. Ecol.Applications 6(4):1002-1017.
Grandin, M. 2009. Can open-ocean mussel farming be lucrative? SeafoodSource http://www.seafoodsource.com/newsarticledetail.aspx?id=4294987485
Gur, N. 1997. Innovations in tilapia nutrition in Israel. Bamidgeh, 49(3):151-159.
Halweil, B. 2008. Fish Farming for the Future: Will Farmed Fish Feed the World? (Worldwatch Report, Sept. 2008. Worldwatch Institute, www.worldwatch.org/node/5881#toc).
Halwart M., D. Soto and J.R. Arthur (eds.), 2007. Cage Aquaculture: regional views and global review. FAO Fish.Tech. Pap. (498):241 pp.
ICLARM (The World Fish Center). 2002. Producing larval sea cucumbers in New Caledonia. Internet: www.cgiar.htm>www.cgiar.org/iclarm/
Isaksson, A. 1996. Ocean ranching: its role and contribution to Pacific and Atlantic salmon fisheries. In: C.V.Voitglander (ed.). The State of the World’s Fisheries Resources, pp. 133-149. Proc.World Fish.Congr., Plenary sessions. 204 pp. (Oxford & IBH Publishing Co.Pvt.Ltd., New Delhi, India.
Issar, Arie S. 2008 Progressive development in arid environments: adapting the concept of sustainable development to a changing world. Hydrogeology Journal, Springer Berlin / Heidelberg V. 16, 6, pp. 1431-2174 (Print) 1435-0157 (Internet).
Issar A. S. 2009. Progressive development to sustain food supply in the arid and semi-arid regions (ASAR). Journal of Arid Environments, Elsevier Volume 73 issue 3. 396–397
Ito, S. and H.Kitamura. 1998. Technical development in seed production of the Japanese sea-cucumber Stichopus japonicus. Beche-de-mer Info.Bull. (10): 24-28.
Jackson, A. 2009. Fish in Fish out rations explained. Aquacult.Europe, 34 (3). Sept. 2009.
Kissil, G.Wm. and Lupatsch, I. (2004). Successful replacement of fish meal by plant proteins in diets for the gilthead seabream, Sparus aurata L. Isr. J. Aquacult. Bamidgeh 56:188–199
Kraft, D. 2007. In the desert, fish farming is a fertile enterprise. The New York Times, 03-0-2007.
Longhurst, A. 2007. Doubt and certainty in fishery science: Are we really headed for a global collapse of stocks? Fish. Res. 2007 (86):1-5.
Moss, S. 2009. An integrated approach to sustainable shrimp aquaculture in the U.S. Clean, Green, Sustainable Recirculating Aquaculture Summit. (Food and Water Watch, Washington D.C.). January 2009
Palawski, D., J. B. Hunn, and F. J. Dwyer. 1985. Sensitivity of young striped bass to organic and inorganic contaminants in fresh and saline waters. Trans.Amer.Fish.Soc. (114):748–753. Internet:
http://dx.doi.org/10.1577/1548-8659(1985)114<748:SOYSBT>2.0.CO;2
Petr, T. (Ed.) 2003. Fisheries in irrigation systems of arid Asia. FAO Fisheries Technical Paper (430). FAO, Rome.
Phyne, J. 1996. Along the coast and in the state: Aquaculture and politics in Nova Scotia and New Brunswick. In: Bailey, C., S. Jentoft and P. Sinclair (Eds.). 1996. Aquacultural Development: Social dimensions of an emerging industry. (Westview Press, Boulder, CO, USA): 281 pp.
Patterson, K. and J.W.Potter. 2003. Proc.Natl.Ac.Sc. 2003, June 25th.
Porter C.B., Krost P., Gordin H. & Angel D.L. 1996. Preliminary assessment
of greymullet (Mugil cephalus) as a forager of organically enriched sediments below marine cage farms. Isr.J. Aquac. 48,47-55.
Purdue News, 2010. Purdue Univ. GMO Fish. Internet information.
http://news.uns.purdue.edu/html4ever/2004/040223.Howard.transgenic.html
Robson, D. 2008. MIT's Self Propelling Fish Farm Prototype (text and video). Internet: Mechatronics; www.NewScientist.com
Rosario, W. 2008. Sea cucumbers in fish ponds. Internet information.
Ryther, J.H., and J.E. Bardach, The Status and Potential of Aquaculture, American Institute of Biological Sciences, Washington, D.C., May 1968, Clearinghouse for Scientific and Technical Information, Springfield, Virginia, Vol. I: PB 177-767.
Saiki, M. K., M. R. Jennings and R. H. Wiedmeyer. 1992. Toxicity of agricultural subsurface drainwater from the San Joaquin Valley, California, to juvenile Chinook salmon and striped bass. Trans.Amer.Fish.Soc. 121:78–93. http://dx.doi.org/10.1577/1548-8659(1992)121<0078:TOASDF>2.3.CO;2
Samocha, T. “Overview of some sustainable, super-intensive microbial biofloc-rich shrimp production systems used by Gulf Coast Research Lab, Waddell Mariculture Center and AgriLife Research Mariculture Lab.” Clean, Green, Sustainable Recirculating Aquaculture Summit. Washington D.C.: hosted by Food and Water Watch. January 2009.
Shapawi, R., Wing-Keong Ng and S. Mustafa. 2007. Replacement of fish meal with poultry by-product meal in diets formulated for the humpback grouper, Cromileptes altivelis. Aquaculture 273, Issue 1:118-126, 30 November 2007.
Sharp, G.D. and C.A.S. Hall. 2007. Neoclassical Economics and Fisheries. In: Leclerc G. and C.A.S. Hall (Eds.). 2004. Making World Development Work: Scientific Alternatives to Neoclassical Economic Theory. (University of New Mexico Press, Albuquerque, USA). 655 pp.
Standal, D and I. Bouwer Utne. 2007. Can cod farming affect cod fishing? A system evaluation of sustainability. Marine Policy ,31(4): 527-534
Tacon, A.G.J. and M. Halwart, 2007. Cage aquaculture: a global review. P. 1-16 in: Halwart M., D. Soto and J.R. Arthur (eds.), 2007. Cage Aquaculture: regional views and global review. FAO Fish.Tech. Pap. (498):241 pp.
IUCN, 2007. Guide for the Sustainable Development of Mediterranean Aquaculture. 1. Interactions between Aquaculture and the Environment and 2 : Aquaculture site selection and site management’ (http://iucn.org/mediterranean -downloadable). The World Conservation Union (IUCN), Gland, Switzerland and Málaga, Spain.
Volpe, J. 2004. Farming is a net-loss proposition -- ecologically, socially and economically. Seattle Post-Intelligencer. January 25, 2004.
Internet: http://seattlepi.nwsource.com/opinion/157539_focus25.html
Zion, B. et al. 2007. Technologies for fish growers. Fish.Fishbreed.Isr. 2007 (1): 1058-1063. (In Hebrew with English abstract).
Zohar, Y., Y.Tal, H.G.Schreiber, C.R.Steven, J.Stubblefield and A.R.Place. 2008. Commercially feasible urban re-circulating aquaculture addressing the marine sector. In: Coasta-Pierce, B., A.Desbonnet, P.Edwards, and D.Baker (Eds.). 2008. Urban Aquaculture., pp. 159-172. (CABI Publishing, Wallingfort, OX, U.K.).
Zohar, Y. 2009. Environmentally compatible, recirculated marine aquaculture: addressing the critical issues. Clean, Green, Sustainable, Recirculating Aquaculture Summit. (Food and Water Watch, Washington D.C.). January 2009.
MARINE FARMING
Perspective on its Inevitability and Sustainability*
“As a primary goal, aquaculture development should conserve genetic diversity and minimize negative effects of farmed fish on wild fish populations, while increasing supplies of fish for human consumption”.
(U.N. Code of Conduct for Responsible Fisheries, 1995).
.
Fish farming or aquaculture was defined by the Food and Agriculture Organization of the U.N. (FAO) as “the farming of aquatic organisms, including fish, crustaceans, mollusks, and aquatic plants”. Marine farming, or marine aquaculture, or mariculture can be defined as “farming of marine organisms”. It also implies some sort of ownership of the cultivated stock (Bailey et al, 1996), although not necessarily of the area over which the marine farm is anchored or staked. Sea or ocean ranching, therefore, doesn’t fall under this definition, since the “seed” fish grown in hatcheries once released are not owned, until captured. Aquaculture, including marine farming, has become a mass provider of animal protein, employment option for poor farmers and displaced capture fishermen, solution for marginal lands and water resources, and as exporter – a foreign currency earner. With marine and inland capture fisheries unable to satisfy a growing demand for some popular fish species, such as for example, salmon, cod and shrimp, as well as trout, tilapia and carps, respectively, it has also become their complementary source.
Aquatic foods have high nutritional quality, contributing on the average 20 percent or more of per capita animal protein intake for almost 3 billion people, mostly in developing countries. Fish is also the world’s most widely traded foodstuff and a key source of export earnings for many countries with particular significance for small island states and, as far as fish farming goes, for countries in the South and Southeast Asia.
History. Aquaculture has been practiced by mankind since times immemorial. Five thousand years ago, Chinese villagers trapped carp in artificial lakes that formed when flooded rivers receded. Ancient practice of carp culture in China was described in the 5th century BC, while the Egyptians might’ve been cultivating fish a thousand years earlier. Also the ancient Romans grew oysters and, most probably, fish, already 2,000 years ago. Around 600 A.D., the Menehune of Hawaii built a huge fish pond on Kauai, as the legend goes, by erecting overnight a 900-foot-long wall out of lava rock, (Cufone, 2008).
Most of the ancient fish farming was based on capture of young stages and transferring them to ponds and sea enclosures to grow. This could be done, for example, by fencing off tidal areas toward ebb tide, which arrested fish movement seaward and facilitated their collection during the outgoing flow. Another method, practiced till the present in some places, is letting fish enter open enclosures during seasonal floods to feed and grow there, and fencing them off before the water recedes. Fish are kept in those enclosures to grow, or collected and moved elsewhere. Such types of culture can be seen even today, for example, in Italy’s “vallicultura” and in SE Asia.
All these and other early variants of fish farming have been of the extensive type. Extensive fish farming implies that fish are not crowded much beyond their population density in nature and are not or almost not artificially fed. Thus, extensive fish farming hardly affects natural habitats. Environmental problems appeared only with intensification of the production.
While the ancient fish farming was based on eggs/larvae/juveniles collected from nature, in a true full circle aquaculture they should be produced on the farming premises. First to close the growth circle was a German farmer in the 18th century who fertilized trout eggs with their sperm and hatched them in tanks/ponds.
During most of the 20th century, marine fisheries and fish farming were developing in parallel as separate industries with little market interaction. By and large, each produced different species and had respective traditional consumers. But during recent decades, things have been changing on the fish market. Now many consumers buy fresh or smoked salmon, sea-bream, oysters, or frozen shrimp, many without knowing, and some without caring, whether they were caught in open sea by fishermen, or grown in ponds, mollusks farms, or floating cages. Presently, farming of some 15 species of marine finfish is in its starting phase or expanding, each according to the progress made by researchers and fish farmers.
Notwithstanding, capture fisheries and fish farming are inter-related and to a great extent overlapping in their ecology, economics and social impacts. Both interact in several manners and often co-exist in common ecosystems. This essay approaches marine farming development, recognizing the fact that our whole civilization has been based on man-modified ecosystems both on land and in water, and that further modifications are required in view of the badly mishandled needs of the expanding human population and the sustained destitution of many.
Statistics. In 2007, the total world fisheries yield was over 140 million mt, of which some 90 million was produced by capture fisheries and over 50 million by aquaculture, most of it in the developing countries, with China leading with some 31 million mt (FAO, 2010). In 2006, the world consumed 110.4 million mt of fish, 51.7 million mt originating from aquaculture. The earth’s population is forecast to reach 8.32 billion in 2030. If capture fisheries production (92 million mt in 2006) and the non-food uses of fish (33.3 million mt in 2006) remain constant, aquaculture needs to produce 80.5 million mt by 2030 in order to maintain the current per capita consumption of 16.7 kg. That is, 25 years from now, aquaculture will need to produce 28.8 million mt more per year than its current annual production (FAO, 2008).
The recent development of aquaculture. During the last 4 decades, fish farming has expanded to meet the soaring global demand for seafood. Both on land and in the sea it has undergone tremendous quantitative development, and soon will be overtaking capture fishery in the global supply of food fish (Tacon and Halwart, 2007).
Consumption of fish, both wild and farm raised, has doubled since 1973, with most of this increase in developing countries. But as the demand for fish rises, wide populations of both marine and freshwater species are unable to produce significantly increased yields, because of stagnant or declining catches. The total marine fish yield has reached 90 million mt a year, a yield that after a period of rapid growth and despite increasing demand has remained mostly level for the last decade.
Since the later years of the 20th century, salmon has become the main finfish farmed in cold waters, milkfish, shrimp, and yellowtail - in South Asia’s and the Far East’s ponds, cages and lagoons, and seabream and seabass - in cage farms all over the Mediterranean. Atlantic salmon, a fish exotic in Chile and on the west coast of North America has been farmed there since the mid 1990s. A relatively late comer to the salmon industry already a decade ago Chile produced more than 100,000 mt/year, and earned in 2006 over US$2.2 billion. This followed a 15-year development during which production expanded by an incredible 2,200 percent. Between 2003 and 2006 Chile’s export earnings grew by an average 22 percent per year, challenging Norway as the world’s top salmon producer.
Unfortunately, it has been suffering during the last couple of years from massive outbreaks of salmon disease that caused considerable decline in production and earnings. Cod farming, already beyond the R&D stage, has become a reality mainly in Norway, the UK and Denmark. Also farming of halibut and turbot is becoming commercial.
The tuna grow-out industry, pioneered in Australia, towards the end of the 20th century has expanded also in the Mediterranean and the Central Atlantic Ocean’s coastal waters. The practice of tuna on-grow (fattening) in cages, however, cannot be considered a real farming. In fact, it consists of creaming off of young fish from the stock years before they had any chance of spawning, and fattening them for half-an-year, or so, before harvesting. This procedure must negatively affect wild stocks of the most sought after tuna species. In Japan, in a procedure that shouldn’t affect wild tuna stocks, it is the fry that is caught to be fattened at a fish farm.
Recently, however, successes were reported of closing the whole life cycle of tunas, i.e., holding breeding stock, making them spawn and fertilize the eggs, on-growing larvae into fingerlings, etc. (Anonymous, 2008; Fiske, 2008). This, if fully implemented could increase very much the production and relieve the pressure on the wild populations. The snag is that tuna are grown on other, forage fishes, and if pellets are used, these would contain high share of fishmeal produced of "industrial", as a rule, small pelagic fishes, of which several kilogrammes are needed to produce one kg of tuna.
The dilemma of growing demand and sustainability. Worldwide, both governments and international and regional organizations define their marine policies as aimed at achieving sustainability in aquatic ecosystems and, particularly with respect to aquatic life and fisheries resources. At the same time, it is widely recognized that the present production of fishery products from the wild and from the existing aquaculture would not be able to match the increasing food needs of the expanding human population.
The U.N. defines…”sustainable development” as development “that meets the needs of the present without compromising the needs of future generations... to be achieved through …balance between environmental integrity, social development and economic development…”. According to FAO, sustainability entails the notion of progressive development, which has no negative effect on the environment and on the future of the resource concerned (Caddy and Griffiths. 1995).
But, “sustainable development” suffers from different, often incompatible interpretations by economists, sociologists, environmentalists, and various stakeholders (Ben-Yami, 2006; Sharp and Hall, 2004). This argument ranges from the extreme “nature-first” conservation approach to a “development and business first” approach. The former is about maintaining of or returning to marine ecosystems as close as possible to their “virgin” or pre-industrial state (Ben-Yami, 1996). The latter is about extraction of fishery resource in the most profitable way, now, (Goodland and Daly, 1996).
Constraints. It is important to bear in mind the various constraints that may impede further development of marine farming.
Although growing sea fish in floating cages may be, under some conditions, less costly than on land, the marine fish farming industry has been focusing on raising species, which when supplied from capture fisheries, fetch relatively high prices. While it indeed may serve as a mass protein provider, its product is intended only for those who can afford the price. Hence, repeating cases of salmon market gluts. They are good and popular fish, but many potential consumers can only afford to pay for them prices that the salmon producing-processing-marketing system cannot live with (Ben-Yami, 1999).
According to FAO, aquaculture would keep growing so that the 2010 world’s total fish production may reach some 140 million mt. While the supplement must come from farming, some 30 million mt of marine fish landings would go for fishmeal needed to feed, apart from poultry and cattle husbandry, and inland aquaculture, all the predatory fishes that are grown in marine cages. This is a nagging problem, all the more that the feed alone may account for some 60% of total production costs (Forster, 2008). Processing and marketing technologies are advancing, and no doubt, some of the today’s “industrial” fish will be made fit for human consumption and fetch prices that would make their reduction to fishmeal uneconomic. Availability of fishmeal, therefore, may become a constraint; hence, quite appropriately, vegetable surrogate feeds are investigated and here and there already applied.
Species that feed at lower levels of the food pyramid, like, carp, milkfish, tilapia (Gur, 1997 and private communication) and gray mullets and, according to information from Israel, also gilthead seabream, can be grown on reduced fishmeal or vegetable diets (Kissil and Lupatsch, 2004). Nonetheless, fish farmers still prefer to use feeds that incorporate some fishmeal, lack of financial motivation being one reason, inertia – another (Ben-Yami, 2004-b). Tilapias are basically freshwater vegetarian fishes, widely cultured throughout the world, with some species showing euryhaline behaviour. Recently Malta University reported on trials aimed at growing tilapia both in sea cages and in tanks with sea water.
Other problems confronting the marine farming industry are environmental concerns, public resistance to genetic engineering, bioaccumulation of various chemical substances by fish grown in polluted waters, frequent outbreaks of stock-devastating diseases, and proliferation of parasites.
Outlook. While markets and consumers get the fish they need, fishing industry strategists and also many fishermen look with wary eye on the ongoing expansion of marine fish farming industry. Although farmed sea fish cannot be sold cheaply, their regular supply prevents wild-fish prices from going up, even off-season to compensate a fishery for poor landings. Together with reduced quotas and subsidies, and several impoverished stocks, this may lead to significantly unfavorable consequences, and even shrinking of the affected capture fisheries. On the other hand, an increase in landings of wild fish of farmed species or market-wise equivalent may affect prices of the farmed fish with negative economic consequences for the farmers.
With this bad news come also a bit better ones. Firstly, where this happens, marine aquaculture may represent a way out for investment capital off the capture industry, and a second line of defense to displaced fishermen by providing employment. Secondly, fish farmed in the sea might resist unfavourable environmental changes in water better than wild fish. This, because if the organisms that form the natural food base of the fish in the wild are seriously affected by such changes, the food base of the wild fish stock would be reduced. Their displacement or disappearance may cause wild population's migration or starvation or both. Farmed fish are supplied with their food whatever is the temperature and salinity of the surrounding water and would suffer only at the extremes of their physiological survival range. All this may make redundant fishing capital and low-income fishing people consider, respectively, investment and employment in the marine farming industry, and in particular – in offshore cage farms, where seamanship, sea legs, boat-operation, and net-making and net-mending skills represent important advantages.
According to FAO, the maximum wild-capture fisheries potential from the world’s oceans must’ve been reached, thus almost any further growth in the world’s fish supply will come from fish farming (FAO, 2006). The rising demand and technological developments that have been fueling the explosive growth of fish farming, have stimulated what is considered by many to be the most hopeful trend in the world's increasingly troubled food system (Hailweil, 2008; Halwart et al. 2007; Ryther and Bardoch, 1968), although targeting at the more affluent consumer (Ben-Yami, 1986). In any case, the mankind is consuming more and more seafood, not so much owing to increase of consumption per capita, but rather due to the continuing growth of the world’s population. In this context both, in terms of demand and of supply, the share of Asian aquaculture is overwhelming.
The commonly proposed solution for the problem of satisfying the demand for fishery products is both to improve the management of exploited fish stocks, and to further the development of commercial fish farming – a process, which is already going on at accelerating pace. There is a continuum between open-access fishing and intensive aquaculture, along which fishing rights and property rights develop from vague to almost absolute (Anderson, 2002), often within shared habitats. However, such developments represent a focus of an earnest dispute on a global scale. This, because they affect inter-related processes within the domain of marine ecology, of economics and of social systems of coastal communities and other people involved in fishing, fish farming and fish processing and marketing.
The spiraling rate of fish farming development, however, has produced also undesirable "side effects” that could have been mostly avoided, would the necessary attention be paid timely and adequate money and efforts duly invested. Notwithstanding, as long as it can produce fish at prices people are ready to pay (Asgard et al, 1999), aquaculture will keep expanding, especially, if wild marine and freshwater yields won't grow, while demand increases.
Feed and energy. Presently, small, forage fish account for some 37% of the world’s sea fish yield, and 25-30 million mt of them are reduced into fishmeal and oil, of which close to half is used for poultry and cattle fodder. In 2006, aquaculture consumed approximately 3.06 million mt or 56 percent of world fishmeal production and 0.78 million mt or 87 percent of total fish oil production with over 50 percent of fish oil going to salmon farming. Between 1992 and 2006 the content of fishmeal and fish oil used in fish feeds has tripled on the expense of the poultry sector, which gradually reduced its reliance on fishmeal. Besides fishmeal or fishmeal-based diets, an approximate 5 to 6 million mt of low-value/trash fish are used as direct feed in aquaculture, and notably in tuna cage culture.
FAO (2008) doesn’t expect any significant increase of fishmeal production, all the more that the fishmeal usage in animal production sector, particularly poultry, will continue to decrease in the coming years. Furthermore, it is expected that a proportion of fishmeal and fish oil used in fish feed will be replaced by vegetable-based protein and oil, and that feed management efficiency is going to be improved. Additionally, price level is bound to affect aquaculture production, especially, when the demand for aquaculture products is outstripping the supply, and fish prices soar so that even inefficient farms make money (ibid; Jackson, 2009).
The expansion of salmon culture in three continents – Europe, North and South America - has resulted in increased demands for feed fishes. Recently, farming of cod – another piscivore – has become the focus for massive development efforts. The magnitude of the farming of piscivorous species competes with the previously predominant culture of tilapia, carp and catfish that utilizes mainly vegetable or algae-based feeds (FAO, 2008).
Apart from the tuna that’s mainly fattened on small pelagics, predatory fish grown in ponds and cages are usually fed with mixed feeds with varying share of animal protein. Fishmeal is very concentrated (some 60% of protein) and normally about 4.5 kg of raw fish are needed to produce 1 kg of fishmeal (Jackson, 2009).
Piscivorous fishes such as salmon and trout must be fed with animal protein; hence fish farming industry has become a major consumer of fishmeal. However, the world resources of fish that can be economically utilized for reduction are finite and as mentioned above, with the right market conditions and appropriate technology, some of them could be used for human consumption and might increasingly leak away to canning, sushi-type and fresh-fish markets. Thus, the presently spiraling growth of aquaculture rather sooner than later must face fishmeal supply constraint.
Altogether, the use of forage fish for fish feed has become a worrying situation. The existing management efforts focus on groundfish and large pelagics, rather than on forage fish, and little is known about their role in the marine ecosystem both as grazers and as food for seabirds and marine mammals, and what the true relation between their natural and fishing mortality is. If and when the demand for forage fish by human food market increases, some of the fishmeal industry and, consequently, also the fish farming industry, may find themselves facing feed prices that may preclude feasible operation. Also, according to Halweil (2008), although raising seafood like oysters, clams, catfish, and tilapia is many times more efficient than cattle breeding, a growing scarcity of fish feed may jeopardize future expansion of farming piscivore fish, like salmon and cod.
If such shift occurs, one would expect increasing reduction of the fishmeal contents in the various feeds and pellets, improving feed-production and feeding-in-cage technology. Today, at a rather conservative feeding coefficient of, say, 4:1, with feed containing 25% of fishmeal, 5 kg of raw fish is needed to produce 1 kg of farmed fish weight. (Jackson, 2009). Only with much higher and hardly practiced fishmeal content of 50%, 10 kg of raw fish for 1 kg of farmed fish weight would be needed.
Reduction of fishmeal content in the feed of some other fishes grown in cages that are feeding in wild on invertebrate fauna, like seabream, is also indicated, in view of satisfactory performance of vegetable proteins (Kissil et al, 2004). Although replacement of fishmeal by vegetable (soy, etc.) meals depends on their respective prices, a steadily increasing amount of the food supply for farmed fish could be coming from soybeans and other vegetable products.
Additional options could be to amplify the preparation of feeds from non-food by-products, such as fishing by-catch and discards, fish-processing offal and fishmeal made of industrial non-food fishes (Alverson et al. 1994), as already up to one-third of fishmeal is made from various fishery wastes (Forster, 2008-b). Another way is using a mixture of poultry by-products and vegetable oils, such as worked out by a Malaysian team headed by Rossita Shapawi (Shapawi et al., 2007).
Claims that those are the fish-farming feed requirements that detract forage fishes from becoming food fish are misplaced, for comparable amounts of fishmeal and oil are going into margarine, cattle feed and chicken feed. Fish farms compete for access to that supply with other, non-fisheries industries, a competition that influences prices for both the fishermen, who catch the small forage fish and for the fishmeal-and-oil industry.
The very processing and transportation of the fishmeal and the forage fish, often overseas, require energy, which reduces the economic efficiency of the whole process. Thus, when the energy costs and other costs of contemporary fish farming in marine floating cages are examined, it turns out that e.g. salmon aquaculture may be even less efficient than extremely inefficient high seas salmon trolling (Folke et al., 1994). On the other hand, the farmed fish consume far less wild fish protein per unit of weight growth than are consumed by wild fish, which must spend lots of energy to chase down their prey. According to Asgard et al. (1999), for example, 10 kg of capelin produces some 4.6 kg of farmed salmon, while the same weight of capelin would only produce 2.0 kg of wild salmon.
Better utilization of both the fish farming area and the energy in the feed would make the industry more environment friendly. But, to introduce and invest in innovative industry practices that may help to create a shift toward progressive fish farming, there’d also be a need for a fundamental change in public attitudes. This includes a willingness to prioritize fish that are lower on the food chain and sensitivity to environment friendly practices. But can consumers today be mobilized to modify the aquaculture industry as they pressured tuna fleets to adopt more dolphin-friendly practices in the 1980s?
Problems. In recent decades, marine farming of seaweeds, invertebrates and finfish has become a main player in national waters of many countries. Therefore, in coastal waters, whether those facing open sea or those in enclosed and semi-enclosed habitats, any fisheries management must cover both capture fisheries and marine fish farming, as it must take into account also all other major stakeholders and participants in activities that affect environment in the managed area.
The "integrated coastal zone management" (ICZM) is supposed to take care of such problematique. Born as a tool to prepare nations for a possible rise in the level of the sea, it is now being applied by governments to harmonise, with their own policies, the often-conflicting interests of the various stakeholders. By definition ICZM has to get involved, at least partly, in fisheries management. The level of its involvement depends on both, the local fisheries, socio-economic and physical conditions, and the respective government policy. (Ben-Yami, 1998; 2005).
Coastal mariculture and in particular fish culture in floating cages, much because of its impact on coastal ecosystems and capture fisheries and on the socio-cultural fabric of fishing communities, have been under substantial pressure of criticism on the part of environmentalist organizations and activists, of commercial and recreational fishermen and other concerned bodies and individuals, and of the ensuing restrictions and regulation. Some, even, like Volpe (2004) insist that the salmon industry is clearly a net-loss proposition, whether viewed from the ecological, social or economic perspective. This criticism cannot be waived aside as that of some of the extreme critics of capture fisheries. It must be seriously examined and considered with the view of finding solutions to the negative effects of the mariculture.
Parasites and diseases spreading in the densely stocked fish cages and coastal marine ponds often cause mass mortalities and great financial losses to the fish farming industry, while escapees from cages may carry them to the vulnerable wild populations. While sea cages protect farmed fish from predators, pathogens can move or be carried both ways. A sea cage thus becomes an “unintended pathogen factory” (Frazer, 2008) or receptacle. According to some reports, each year around two million farmed fish escape in the Atlantic. Of fish farmed, unlike the quantitatively less important wild seabass and seabream, cod and to some degree salmon create large wild stocks of major commercial importance. Hence, the capture fishery is looking with a wary eye on the impact of infected runaway fish on their wild brethren, and on the possibility of interbreeding of farmed varieties with wild stocks. Also, sea-lice spreading from cages to wild fish may increase their lice burdens and cause wild fish to decline (ibid).
While fish infected in the cages have better chance to survive, owing to veterinarian care and various treatments, wild populations are more vulnerable. Massive infections of wild fish can be avoided only by ensuring that they do not share water with farmed fish, either by locating sea cages very far from wild fish migrational paths and feeding/spawning grounds, or through the use of closed-containment systems (ibid).
Fish farms with tons of feed being released into the cages create algae-promoting conditions similar to pollution from cities and terrestrial farms. The situation is reversed with oyster farms, because oysters are filtering animals. These farms provide water-cleaning benefits and support coastal economies. They also make better oysters: a farmed oyster is plumper, sweeter and prettier than its wild cousin. Hence, farming planktivorous, herbivorous and omnivorous freshwater fish like grey mullet, tilapia, carp and catfish, and mollusks like oysters and mussels is environmentally sounder. But the money is in the piscivores, like tuna, salmon and cod. The need to feed them with small whole fish or fishmeal is putting pressure on populations of wild forage fishes, which makes the fish-farming industry competing with wild carnivores over their limited resources.
With time, more problems materialized that, while not markedly slowing down the development, produced negative effects. Such has been, among others, the denuding of mangrove areas by the expanding shrimp farming in Latin America and in the south and southwest Asia, which has allegedly affected the catches in the adjacent artisanal fisheries and their communities, and more recently, was blamed for at least part of the damage caused all over the Indian Ocean by the 2004 tsunami.
Poorly run land-based fish farms, floating fish cages, and feeding pens release into the environment unutilized protein, nutrients and fecal matter creating wasteful and often noxious pollution. According to some estimates, a fish farm with 200,000 salmon releases nutrients and fecal matter roughly equivalent to the raw sewage generated by 20,000 to 60,000 people and Scotland's salmon aquaculture industry produces the same amount of nitrogen waste as the untreated sewage of 3.2 million people - just over half the country's population (Halweil, 2008). Residual feed and bio-products pollute protected waters causing increased turbidity, and over shallow bottom - desertification. Complaints on the part of bathers and the tourist industry have forced some cage farms in the Mediterranean and the Red Sea to transfer their operations ashore (Ben-Yami, 2002).
Effect of fish diseases and other environmental problems on cage farming. Concentration of fish farms both, land-based and marine, along coastline, especially in or near enclosed or semi enclosed marine areas as for example, Norwegian fiords, Scottish lochs, Southeast Asia, China and Japan coastlines, and North American estuaries, is allegedly harming the coastal marine habitats and spreading diseases and parasites to wild fish populations, of which salmon seems to be particularly hit.
Parasites are a serious problem associated with densely populated fish cages. Caligid crustaceans, such as sea lices Lepeophtheirus salmonis and Caligus spp, are parasitic copepods that attach themselves to fish, marking their hosts with ugly lesions that lessen their market value, stunt their growth and weaken their resistance to diseases. Found on wild fishes, they are now common among Atlantic salmon grown in cages in Norway, Scotland, Chile, and North America. But now there’re serious concerns that cage farms of salmon set on the migrational routes of wild salmon increase the risk of infection of the latter by sea lice originating from the fish cages.
The most harmful disease, spreading over cage farms affected by sea lice, is the infectious salmon anemia, or ISA. A highly contagious virus, ISA can be lethal to fish although it does not affect humans. The ISA virus is fast to spread over whole regions causing heavy financial losses and reduction of salmon production. One consequence is operators having to shut farms and processing plants and axing jobs. Also, diseases may leak from marine cages and pens and spread to wild populations – (Diamant et al., 2007). ISA was first discovered in Norway in 1984. In mid-2007, it turned up in Chilean waters, and within 2 years devastated the country's farmed salmon industry, which was forced to curb production by half and shed up to 4,000 jobs.
Another concern is the residual materials from the drugs used by fish farmers to fight parasites and diseases and the pesticides used in agriculture. Their amounts may be substantial in view that, apart from the residual veterinary products in the agricultural effluents flowing into the seas, the fish farming industry alone spends on drugs an estimated $1 billion a year. All those, including toxic substances affect inshore and coastal fish, including the farmed ones, in various ways, sometimes causing various distortions and growth arrest in juveniles growing in polluted waters (Saiki et al, 1992; Palawski et al, 1985).
Anthropogenic pollution and effluents affect both wild and cultured species inshore and in coastal waters. Serratia marcescens, bacteria commonly found in the guts of people and animals is killing off corals and can also kill fish (Patterson and Potter, 2003).
Unpredictable and uncharacterized impact of climatic, weather, and tectonic events presents unquantifiable threats. The first decade of this century has seen unprecedented impacts on aquaculture in Aceh (Indonesia), Bangladesh, China and Myanmar, which have all suffered from severe natural disasters (FAO, 2008).
On the other hand, fish farmed in the sea might resist environmental changes in water, including the effects of climate change, such as temperature variations, better than wild fish, which depend upon their natural forage organisms. Forage species, however, may be more sensitive to such changes than the fish themselves. Their scarcity or disappearance may cause their predators’ migration, starvation or both. Farmed fish are supplied with their food whatever is the temperature and salinity of the surrounding water and would suffer only at the extremes of their physiological survival range.
Aquaculture and the capture fisheries. Allocation of marine areas for fish and shellfish farming had become a problem when farms were established within traditional fishing grounds. While fish cages shouldn’t be and need not be placed over prime or traditional fishing grounds, not everywhere regulations prevent ill-conceived offshore aquaculture projects and provide for their removal. The accelerated development of aquaculture is calling for specific regulation and for allocation of marine areas where fish farms can be established, which willy-nilly must affect some traditional and other fisheries, through redistribution of access to fishing grounds essential for setting-up of the fish-farms. Notwithstanding, some of the claims regarding displacement of fishing grounds by aquaculture seem exaggerated, at least when it comes to open sea. The area taken by fish farms over exploitable fishing grounds is significant fishing-wise in a relatively few cases.
Apart from complaining that marine fish farms are expanding over fishing grounds, many commercial fishermen, oppose fish farming in floating cages, because of the associated influx of nutrients into the environment and of biological effects of densely populated fish farms on the habitat and wild stocks through broadcasting of parasites and viral diseases over wild fish populations and possible genetic effects due to massive escapes of farmed fish into open sea. The possible effects of fish escapes from cages in areas where massive marine farming is practiced, as in the N. Atlantic, at both sides of the Pacific, in Chile’s waters and in the Mediterranean, have been widely studied and discussed (Ben-Yami, 1999; Dempster et al., 2007; Halweil, 2008; Standal and Bouwer Utne, 2007).
All this taken into account, growth of supply of fish from marine and on-land farming is a must, as long as mankind keeps its excessive proliferation and the demand is growing, and properly managed fish farming can not only help feeding an expanding global population, but also relieve the pressure of fishing on wild fish populations (Halweil, 2008).
PROSPECTIVE DEVELOPMENTS
The question is not how to prevent aquaculture from harming the marine environment, but how to develop aquaculture that’s environment friendly.
It is beyond question that, in spite of its shortcomings and contention with other stakeholders, marine fish farming is here to stay, develop and expand. Restraining its growth is not in the best interest of the protein-hungry mankind, and any such attempts, including those flying the banner of sustainability, will eventually prove futile. The world is in need of a progressive development approach (Issar, 2008 & 2009) that would focus on how marine fish farming could expand while mending its ways, so that its present and future structure and development would cause minimum environmental and social damage and maximum social and economic benefit.
Regulation. For this purpose, it must be recognized that regulation of marine farming both, by national authorities in coastal waters, and by international agencies in the open ocean is crucial. The choice of technology and culture methodologies cannot be left to private enterprise alone, for it would obviously be looking for the most profitable, rather than for the most environmentally and economically feasible techniques. The same goes with respect to the contaminants content in the farmed fish flesh. This points to a greater role for aquaculture certification and standards and to labelling, such as exist, for example, in organic and local foods sector (Halweil, 2008). Progressive regulation should force the marine farmers to design and apply ways and means that while maintaining economical feasibility would be environment friendly.
Unfortunately, legal mechanisms available to legalize and regulate ocean fish farms in national and international waters are few and far between. One problem is that different national agencies have jurisdiction over different aspects of activities in seas and oceans, such as commercial shipping lines, fisheries, naval restrictions, coast guard and safety issues, pollution control, sand end minerals dredging, oil and gas explorations, offshore wind-energy farms, and extraction facilities like rigs and pipelines, etc. Many countries have not yet decided on specific standards for establishment and managing offshore fish farms, and/or on which agency or agencies should govern such fish farms, and how.
All this can make regulation, including site allocation and licensing, as well as enforcement, a highly controversial political issue (Bailey et al, 1996) with conflicting stakeholders’ interests, especially with regards to small private and community-operated enterprises versus corporate interests, and fish farming versus (commercial and recreational) fishing interests. On the top of it are powerful environmental lobbies that, rightly or wrongly, partly or totally, resist mariculture. Therefore, the very existence and character of state policies or their absence, as well as the level at which they’re implemented are critical to the development of marine farming, (Phyne, 1996).
Much of the conflicts could be avoided, if mariculture developers would follow such procedures as those appearing in the 303-pp ‘Guide for the Sustainable Development of Mediterranean Aquaculture 2 (Ben-Yami, 2010, IUCN, 2007).
Notwithstanding, marine aquaculture will keep developing, within the existing legal frame, or if non-existent, unrealistic, or ineffective – without. The extent and character of this development would be in accordance with the extent and character of market demand, while adjusting to its changing preferences, such as growing demand for organic products, attention to environment-friendly production procedures, etc.
TECHNOLOGY
Storm-proof cages. Submergence represents the most logical solution to assuring survival of fish cages and tanks in stormy weather, and is a must in case of net cages that are not fitted in stiff framing. The main principle of the submersible cages technology is that upon the approach of stormy weather, the cage is submerged, desirably by remote control, to a safe depth at which it is not affected by sea waves when the sea is rough. Several variants of submersible cages have been designed and some are in use, their shortcoming is that to be effective, they are not built as large as some of the surface-floating ones.
Some fish species suffer from various forms of decompression sickness, if they’re forced to ascend too fast from a deepwater submergence, cod, for example, may burst its air bladder. Therefore, the ascent of submergible cages containing such fish up to the surface should be quite gradual. This technology would keep improving and developing towards automatic submerging and surfacing of the cages by means of weather sensors and remote control. Another solution is to keep the cages permanently submerged and serviced by automatic feeding systems, and making them surface only for harvesting (Forster, 2008).
The special needs of servicing, mechanization and automation in marine fish farms would bring about design, vessel conversions, and construction of specialized sea-going work-boats (ibid), with size, form and functions fitting the design, location and character of the individual fish farms and their cages.
Containment. Closed-containment technology can prevent escaping into the ocean of both, fish that may affect wild stocks, and water that would spread parasites and thus also diseases. Closed containment farming requires floating tanks that must combine ship-type strength and seaworthiness with maximum mechanization and automation of servicing by remote control. They may be anchored or secured to piles, or even free floating in oceanic currents (see “Ocean drifters”, below). Fish in containment tanks won’t be able to escape and would grow without risk of attacks by marine mammals, which is common in regular net cages, while filtrating or other pollution preventing system would minimize environmental effects.
Abandoned oil rigs, artificial reefs and islands. One idea is to develop finfish aquaculture industry using open net pens or submersible cages in association with derelict oil rigs. Around such rigs, as with any offshore natural (reef, sea-mount) or man-made structure, would develop a multi-species biotope, including micro-fauna and other planktonic organisms that might serve as extra food for the fish in cages. Artificial islands and their immediate surroundings (Ben-Yami & Nir, 1990) could serve as bases and sites for marine fish farms. Seeding bio-filtering organisms to create their colonies/reefs in the vicinity of cage farms can reduce the deterioration of water quality due to waste and residual food from the cages (Ben-Yami, 2004).
Expansion of aquaculture over additional species. Dried sea cucumber fetches extremely high prices on the growing Chinese affluent markets as a delicacy and an aphrodisiac. So far, all the supply of sea cucumbers has come from collecting from the wild inshore and in shallow coastal areas, which brought about substantial impoverishing of the resource. Efforts towards stocking and even farming sea cucumbers have been going for years (Ito and Kitamura, 1998; ICLARM, 2002). Recently, in the Philippines sea cucumber was cultured for the first time in fishponds in polyculture with shrimp, following a successful experimental breeding that produced juveniles for stocking both, in fish farms and in the wild (Rosario, 2008). In the future, more and more commercially valuable finfish and invertebrates will be cultured, following the ongoing research and experimentation.
Polyculture. Fish in cages utilize the energy of only part of their feed. The remainder and the waste are flushed out of the cages. Hence, the idea to cultivate species that live at different stages of the food chain on the same or neighbouring site. For example, keeping salmon, mussels and kelp in the same system may enable the feed to be fully utilized, because the mussels and kelp can absorb it in various ways and grow on the feed not consumed by the fish and their waste (J.N.Galloway – Internet). Another solution could be keeping non-fed omnivorous fish in the lower level of “double-bottom” cages, where they can feed on what’s sinking through the “floor” of the upper level where carnivorous fish are intensively fed (Porter et al, 1996).
Genetic engineering. Genetically modified organisms (GMOs) represent a controversial issue in agriculture. Significant research has gone into producing GMO fast-growing fish and transgenic aquatic organisms, and some are ready to be placed on the market and under the U.S. Food and Drugs Administration’s (FDA) scrutiny (NY Times, June 25, 2010). However, the global debate on their possible risks to human and environmental health and in part inspired by moral values, sometime rather sanctimoniously, is continuing at such a scale that, according to FAO (2008), it is unlikely that in the short term GMOs will make a significant contribution to meeting the future demand for fish (Purdue News, 2010; The Center for Food Safety, 2009).
Farming for bio-fuel production. One way in which marine farming can do good service to the global environment is to grow marine plants, including algae, for bio-fuel production (Forster, 2008-b). Since this could be done on the basis of waste, effluents, and other coastal pollution, on which the plants would grow, the total environmental benefit should be considerable.
Aquaculture off-shore. There are many reasons why the marine farming sector is aiming for the open sea, in spite of some claims that open net cages flush pollution, disease and parasites into the ocean, adversely impacting wild fish supply, and the health and sustainability of the oceanic habitat. However, the water quality here is better and the flow rate is higher than within narrow or partly enclosed inshore areas, and the temperature is more stable – factors that improve the growth conditions and the quality of the fish flesh. Open water systems farther offshore should be less of a concern, because the waste dilution process is more efficient, so that cages sited over deep ocean water, especially where oceanic currents prevail, may have little impact on the surrounding water quality or the ecosystem, in general. Also the available evidence suggests that in open ocean aquaculture there is no measurable nutrient loading in the effluent. However, offshore aquaculture creates also its specific set of complications, such as problems with rough weather conditions, allocation and enforcing farm siting rights, and servicing the cages far offshore.
The pro-and-contra dispute is ranting, and in view of the evident necessity for further expansion of marine farming, people are expecting national and international regulation, and are looking at technology. Unsurprisingly, some rather futuristic ideas have popped up.
Open-ocean mussel farming
A new pilot-project run by the Marine Biological Laboratory (MBL) in Woods Hole, Mass., and coordinated by Scott Lindell, launched four experimental mussel farms to test blue mussel farming offshore Massachusetts and Rhode Island. It uses technology developed at the University of New Hampshire, consisting of anchored longlines suspended 10 m below the ocean surface, holding biodegradable “socks” filled with mussel seed, and relies upon the oceanic currents for the supply of food to the growing mussels. This development may lead to the creation of a multi-million dollar sustainable industry involving local fishermen, existing shore-side infrastructure and an underutilized natural resource.
Ocean drifters. A team at the Massachusetts Institute of Technology reported designing a giant remotely operated motorized cage, which is supposed to be carried by ocean currents, in a controllable manner. The idea is to keep cages drifting within a limited area or along a desired path, so that they can be filled with fingerlings, for example in Florida, and drift in the Gulf Stream for nine months to reach Europe with a market-sized crop. The MIT’s drifter-cages would be fitted with low-power hydraulics or electric self propulsion to allow them to remain within the current and stay away from bad weather. Not being anchored over a fixed spot, there’ll be little benthic impacts. MIT has just completed in Puerto Rico the first round of experiments with the Self Propelling Fish Farm Prototype. One of the research scientists’ visions is an advanced fish farm that can “think for itself”. Such fish farms will be able to float to more optimal locations when required and submerge in the sea when exposed to rough weather. Even though this vision is unlikely to be a reality for at least 10 or 15 years, most of the technology for fish farms drifting in the open sea is already available (Robson, 2008).
Sea ranching. The term sea or ocean ranching is used here to describe the attempts at increasing the output of natural and fishery ecosystems through the stocking of juveniles into vast open areas (Isaksson, 1994), but mostly in inshore and coastal waters. Such stocking with fingerlings or juveniles of edible invertebrates and finfish is limited to species that are either strictly local or seasonally returning to the locality, and depends on aquaculture technology that controls spawning and/or egg and larval development, until the animal grows to a stockable size.
Culture and stocking of juvenile lobsters and sea cucumbers in the coastal wate+++rs of Galapagos was proposed as a way to conserve the islands’ ecosystem, in view of heavy extraction of these species by local fishermen (Ben-Yami, 2001).
A group of Israeli fish farmers and scientists put forth an idea of fish ranching, which they call “virtual cage technology for farming of fish”. They propose to train juvenile fish in hatcheries to associate acoustic and visual signals with food. Trained fish are released to the sea to grow in nature; however, to perpetuate their response to the signals they’re fed periodically from floating platforms. At harvest time the acquired response would attract the fish to collection sites to be trapped and harvested selectively using a computerised vision system. This technology offers an alternative to cage farms and is almost neutral environmentally. It should be beneficial to fishermen and consumers, and may complement coastal cage culture and in some places replace it. It would save investment in expensive offshore cage farms and in floating growth-and-service installations and feeding technology, and save on running expenses for servicing labour (Zion et al, 2007). This, rather futuristic development requires working only with fishes that tend to stay in one locality, grow fast enough in nature, and have a good value on the market.
MARINE FISH FARMING ON LAND
Carrying sea fish farming ashore opens several interesting options. One is to regard the fish to be only one component of an integrated system in which also other organisms are grown. Such aquaculture could be based on algae or other plants, and designed to develop in a way, which could exert less pressure on the environment (Forster, 2008-b) and even enhance it. In addition, the option to grow sea fishes in land-based installations or in ponds represent a solution for situations where sea-borne farming in cages is excluded for environmental reasons or due to successful pressure of other stakeholders (Ben-Yami. 1999; 2002).
Closed, integrated systems. A promising development is integrated
aquaculture, for example, growing seaweed, salmon and mussels in a single installation. The seaweed and mussels live and grow on the waste from the fish. This principle may be further integrated in a closed system where the fish are fed and produce nutrient-rich waste. Solid fish waste may be removed to feed, for example, worms bred for bait. Water with dissolved nutrient waste may then be piped or otherwise released to feed water-filtering shellfish and plants. Such system can be fully closed as far as fish are concerned with very little additional water needed and no waste released (Moss, 2009).
Dr. Yonathan Zohar developed at the University of Maryland fish farming technology for the urban environment. His special self-contained and densely populated fish tanks are filled with recirculating water, kept free of waste by bacteria cultured on plastic plugs. Fish swim in tanks in water free of nutrient waste and are not exposed to toxic contaminants and poor water conditions. They can grow faster — eight to nine months compared with 14 to 16 months — and at much higher densities. The toxin-free fish can be marketed as clean, and conditions can be tailored to fit the fish. Farming sea fish in such land-based closed systems may also prove economically more feasible than in floating cages in open sea (Zohar, 2009; Zohar et al, 2008). On the other hand, however, important elements of this technology may well find applications also in marine fish farms where closed containment fish tanks are employed at sea. Most recently, such self-contained super-intensive shrimp culture system was developed at Texas A&M by Dr. Tzachi Samocha, (2009).
Fish farming in arid areas. In some arid and semi-arid areas, as for example in Southern Asia (Petr, 2003), the Middle East (FIS - Newsletter <newsletter@fis.com> Oct 04 2007) or in the Israeli Negev, saline, often warm ground water is available. While such water may be too salty to support agriculture, it may be suitable for aquaculture, starting with algae of species valuable for diverse end uses, including medicinal ones, and ending with marine and euryhaline invertebrates and finfish (Kraft, 2007). Development of such aquaculture may become an effective alternative livelihood for people living in arid areas. Undoubtedly, any such development would require substantial investments in research and infrastructure, choice of or devising methodology and, because of the need for strong technical support - an area-wide extension service. Studies and experimental culture projects have yielded a range of marine and freshwater finfish species that can be successfully grown in the desert, including tilapia, barramundi, sea bass, striped bass and red-drum.
In recent years this option has been attracting attention of international organizations, and quite rightly so, in view of the fact that semi-arid and arid areas constitute more than 40 percent of the global land area. Such areas are home to nearly one third of the global population, the bulk of who live in developing countries and represent some of the poorest people in the world. ‘Arid aquaculture’ in dry lands would present a supplement or a substitute to traditional crop farming and livestock rearing, might mitigate the consequences of desertification and improve nutrition through diet diversification.
CONCLUSIONS
General
Both, in spite and because of the fact that capture fisheries and marine farming in some areas are sailing on collision course and in others in a shaky and often volatile co-existence, the future development and management of both industries should be comprehensively designed, planned, and executed within the context of ecosystem management. Both industries are essential and even crucial for supplying the humanity with protein, in view of the pressures of the ever increasing demand. Constraining mariculture development is a non-starter, for it must lead to obstructing growth of fish supply and even to reducing fish production. Therefore, the easy way out of trying indiscriminately to curb their future development and even to cut down both, under the banners of “sustainability” and "precautionary principle" is unsustainable. Nonetheless, such course of action is assisted by alarmist and fallacious "scientific" articles published only due to inadequate peer-review process (Ben-Yami, 2003; Longhurst, 2007), and hence propagated by the general media always happy to quote sensational "science”.
A progressive approach (Issar,op.cit.), therefore, must be adopted and implemented for the development of marine aquaculture so that it is environment-friendly and socially acceptable, and that it can live in sustainable coexistence with marine capture fisheries within their common ecosystem, benefit fishing people and other fishworkers, and not less important, the consumers. The following conclusions can be suggested:
(1) It is imperative that, for the sake of sensible, rational and efficient management, both industries, marine aquaculture and capture fishery, fall in their shared countries under the same legal and enforcement authorities. This will enable rational allocation of farming sites and fishing grounds, abatement of conflicts, and maintaining an equitable modus vivendi.
(2) It is important to recognize that there’re no “one-size-fits-all” solutions, and there’s no panacea for every problem encountered by fish farmers, fishermen, and authorities in charge of fisheries (capture and aquaculture) management throughout the world. Every environment, every fishery, every fish species, and every site impress their specific conditions and requirements.
(3) Notwithstanding, a general approach in the spirit of progressive development should be common to all marine fish farms and, for that matter, the whole mariculture industry. While such approach takes for granted the need for and inevitability of further expansion of marine fish farming, its future management and development should be based also on the following principles:
(3.1) farm sites should be selected and allocated so that they do not take up traditional fishing grounds, especially those of small-scale and subsistence fishermen;
(3.2) farm sites should be selected and allocated so that they are not on the way of massive, seasonal or annual, migrations of wild fish populations;
(3.3) marine fish farms should be operated according to clear and strictly enforced rules aimed at minimizing their effect on the neighbouring habitat;
(3.4) marine fish cages should be designed to prevent escapement of fish and, especially in shallow waters, to contain or re-cycle residual feed;
(3.5) application of drugs and chemical substances, such as pesticides, hormones and antibiotics, should be regulated, monitored and the ensuing rules strictly enforced;
(3.6) coastal aquaculture farms should not be established upon environmentally sensitive, in particular mangrove-covered coastal areas;
(3.7) marine farms should not import and breed exotic species, except if established by specific research that their eventual escape into the wild would not be detrimental to the local biota and ecosystem;
(3.8) fish farmers should, as far as feasible, minimize the use of small forage fishes and fishmeal made of them.
(3.9) in fishery management, the "precautionary principle" should not be blindly applied with "fish first", whenever the managers find themselves in doubt as to what to do. It should be borne in mind that the resource is managed for the sake of people, and that the "doubt" in the expression "when in doubt" may still carry a tendency to lean either in favour of the resource or of the humans, and that the " taking no risk whatsoever" strategy may be detrimental to human societies;
(4.5) ecosystem management must pay the due and appropriate attention to reduction and elimination of all sorts of pollution and its sources, to prevention of inshore habitat destruction and to recovery of essential habitats for fish reproduction and nurseries, such as mangrove forests, wetlands, reefs, etc. On the other hand, it must take into account changes and fluctuations in marine climate, its physical and chemical phenomena, and the various biological factors that affect recruitments and natural mortality in wild fish, and to a lesser extent in the farmed ones..
***************
The needs of the world population, the constraints of the wild fishery resources, and the new problems arising in the wake of the development of marine farming, require a major shift in the prevailing interpretation of the term sustainability and, hence, in the approach to the management and development of all sectors of marine fisheries. The gist of the new and progressive approach is to fulfill two main objectives: 1 – increasing the supply of marine products to the people; 2 – sustain the fishery ecosystem on all its components: the producers and their communities, the fish and their habitat, and clean water in the sea.